KEY POINTS
- Appropriate hydration practices during exercise are important for avoiding fluid-electrolyte imbalances and maintaining performance.
- Because of the considerable variation in sweating rate and sweat electrolyte concentrations, personalized fluid replacement strategies based on individual sweat profiles are recommended.
- Sweat testing has not been widely available for everyday use outside of elite sports and clinical research settings.
- GSSI recently developed a wearable microfluidic device (Gx Sweat Patch) and smartphone image processing platform (Gx App) for on-body measurement of local sweating rate and sweat chloride concentration. Algorithms in the Gx App are used to predict whole-body sweating rate (WBSR) and whole-body sweat sodium losses from the local measures obtained from the Gx Sweat Patch.
- The Gx Sweat Patch and App enable individualized sweat testing under field conditions with no need for specialized expertise or laboratory tools.
- The Gx Platform has been validated against standard sweat testing methods, including the mass balance technique for WBSR and whole body washdown for sweat chloride and sodium concentration.
INTRODUCTION
Appropriate hydration practices during exercise are important to avoid fluid-electrolyte imbalances and help maintain performance (McDermott et al., 2017; Sawka et al., 2007; Thomas et al., 2016). The key factor impacting fluid intake requirements is sweat production, and it is well known that there is significant inter-individual variation in sweating rates (~0.5-2.5 L/h) and sweat sodium and chloride concentrations ([Na+] and [Cl-] ~10-90 mmol/L) during exercise (Barnes et al., 2019). Insufficient fluid intake in the face of high volumes of fluid loss through sweating can increase the risk for significant hypohydration (≥ 2% body mass loss) (Belval et al., 2019). On the other hand, overdrinking relative to sweat losses increases the risk of developing hyponatremia, particularly during prolonged events (Hew-Butler et al., 2015). Individualized sweat testing is recommended to help identify athletes with a higher versus lower risk of significant fluid and electrolyte losses (McDermott et al., 2017). However, sweat testing can be an involved process, requiring certain specialized equipment and expertise to analyze and interpret the results. In particular, sweat composition testing is not widely available for everyday use outside of elite sports and clinical research settings.
Whole-body sweat losses are typically estimated by measuring change in body mass before and after exercise while also accounting for any fluid intake and/or urine loss during the test session (mass balance approach) (Cheuvront & Kenefick, 2017). The reference technique for whole-body measurement of sweat [Na+] and [Cl-] is the laboratory-based washdown procedure (Lemon et al., 1986; Shirreffs & Maughan, 1997). A practical alternative approach is to predict whole-body sweat losses from local sweating rate (LSR) and local sweat electrolyte concentrations (Baker et al., 2018). Still, assessing sweat profiles using established local sweat collection and analysis techniques is a slow, labor-intensive process. While the absorbent patch technique has been widely used with athletes to measure sweat electrolyte concentrations (Barnes et al., 2019; Maughan et al., 2004), the required post-collection harvesting and expensive benchtop analysis of sweat is impractical for the general population and precludes real-time feedback. Advances in wearable technologies have enabled a simpler, less intrusive approach to sweat testing. To this end, the Gatorade Sports Science Institute (GSSI) recently developed a wearable microfluidic device (Gx Sweat Patch) and smartphone image processing platform for on-body measurement of LSR and local sweat [Cl−], as well as algorithms to predict whole body sweating rate (WBSR) and whole body sweat Na+ losses (Gx App) (Figure 1) (Baker et al., 2020). The purpose of this Sports Science Exchange article is to briefly describe the science behind the Gx Platform and its practical utility in facilitating personalized fluid intake recommendations.
Gx SWEAT PATCH
Overview
The Gx Sweat Patch (Figure 2) is a flexible stack of thin-film polymeric materials (3M) consisting of an enclosed network of microchannels. The patch collects sweat by a process called microfluidics, as the channels in the patch route sweat by exploiting the natural pressure associated with eccrine sweat excretion. It adheres to the skin via a patterned hypoallergenic adhesive integrated into the bottom surface of the patch. Inlet windows define two distinct sweat collection regions interfacing with the skin, including one for LSR and another for sweat [Cl-].
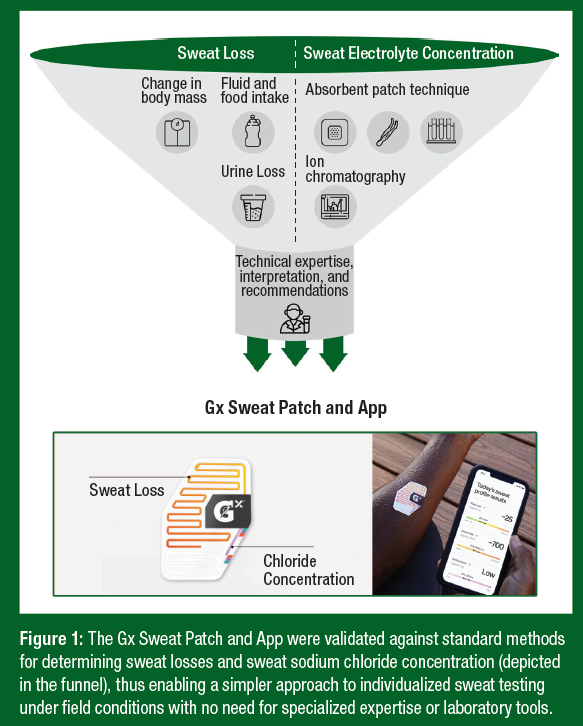
Microchannel 1 contains an embedded orange dye that mixes with excreted sweat near the inlet so that propagation of sweat is visible as it flows through the channel pathway. The front of the orange streak can be measured to determine collected sweat volume over time (LSR). Microchannel 2 contains silver chloranilate used for colorimetric analysis of local sweat [Cl-]. Sweat entering Microchannel 2 mixes with the chloranilate, producing a purple color with an intensity that increases monotonically with [Cl−]. It is important to note that chloride is the electrolyte of choice for the microfluidic patch because the colorimetric chloride assay is well established, and the chemical reaction is robust at various ambient temperatures. By contrast, sodium assays are less stable, and none exist that precipitate sodium for quantitative measurements via colorimetry. However, based on work from our laboratory (Baker et al., 2020), there is a strong correlation between whole-body sweat [Cl-] and [Na+], allowing accurate predictions of sweat [Na+] (Figure 3).
Instructions for Use
Applying the Gx Patch to the Skin.
The Gx Sweat Patch should be applied to the left ventral (inner) forearm, about 2-3 inches below the elbow crease, prior to exercise. For optimal patch adhesion, the skin needs to be cleaned (e.g., with an alcohol wipe) and allowed sufficient time to air dry prior to application. It is critical that the forearm is free of skin-care products (lotions, sunscreen, etc.) before patch application. In addition, the user should firmly press down for ~5- 10 seconds to seal the patch onto the skin. The ventral forearm was chosen as the regional site because of the significant correlation with WBSR and whole-body sweat electrolyte concentrations (Baker et al., 2018; 2019). This location is also convenient for users to photograph with their smartphone and tends to have less hair than other sites. Shaving is not necessary for most individuals but may be helpful for adhesion if hair follicles are dense. The Gx Sweat Patch can be placed on tattoos (see Research Validation section below for more details).
Obtaining Sweat Profile Results.
To obtain sweat profile data the athlete or practitioner should use the Gx App or Gx Teams (see description of each below) to take an image of the Gx Sweat Patch while it is still on the skin. The image should be taken after completion of the exercise session or just before the orange channel fills completely during exercise, whichever comes first. The minimum wear time to obtain sweat profile results (i.e., until colored sweat is visible) varies depending on the athlete’s sweating rate. Individuals with low sweating rates (e.g., LSR of ~0.5 mg/cm2/ min) may need to exercise for at least 60 min to obtain results. Heavy sweaters (e.g., LSR of ~2.5 mg/cm2/min) could see color changes in the patch within 20 min, while the maximum wear time before the channels overflow could be ~60-70 min.
The Gx App on a smartphone processes the results to determine the user’s sweat profile (i.e., whole body sweating rate, total sweat loss, and sweat Na+ loss). Personalized fluid intake recommendations are provided based on the user’s sweat profile. The user would then follow the recommendations to properly rehydrate immediately after the workout and/or properly hydrate during their next workout of similar intensity, duration, and environmental conditions. The sweat profile results and recommendations are provided as a range (prediction interval) to account for inherent day-to-day variation and error in the prediction models.
Gx SWEAT PATCH VALIDATION RESEARCH
A series of four large clinical trials (Table 1) were conducted to compare Gx sweating rate and sweat [Cl-] with the absorbent patch technique and to develop algorithms to predict whole-body sweat profiles. Consistent methodology was used in each study. Sweat was collected from the right and left ventral forearms with an absorbent patch (Tegaderm+Pad, 3M; pad size 11.9 cm2) and the wearable microfluidic patch, respectively. This was considered a valid comparison since the literature suggests there are no significant bilateral differences in forearm LSR and sweat electrolyte concentrations (Baker et al., 2018; Dziedzic et al., 2014; Morris et al., 2013; Verde et al., 1982). Absorbent patch LSR was measured using gravimetry. Local sweat [Cl-] and [Na+] were measured via ion chromatography. WBSR was measured using the standard mass balance technique, corrected for urine loss, fluid/ food intake, metabolic mass loss, and respiratory water loss. The whole-body washdown technique was used to measure whole-body sweat electrolyte concentrations (Study 1 only) (Baker et al., 2020).
Table 1 provides a brief description of the four validation studies and a summary of results. The first study (Baker et al., 2020) demonstrated significant correlations between the Gx Sweat Patch and the standard absorbent patch technique in measurements of LSR and local sweat [Cl-], both in a controlled laboratory setting and in the field. In addition, the coefficient of variations (CVs) for the microfluidic device were similar to that of the reference techniques for both LSR (9%) and local sweat [Cl−] (12-13%) (Baker et al., 2020). These CVs are also consistent with previous research investigating day-to-day variability in forearm LSR and electrolyte concentrations (Baker, 2017). In addition, models were derived to predict WBSR and whole-body sweat [Cl-] from microfluidic regional data. In total, 312 athletes (194 males and 118 females; 15-45 years) participating in a range of indoor (stationary cycling, basketball) and outdoor (soccer, tennis, lacrosse, track and field) sports were tested in temperate to warm conditions (21-35°C, 25-82% relative humidity) in this original study.
Since the first study was conducted mostly with competitive athletes in warm conditions, the objective of the second study (Baker et al., 2022b) was to test the validity of the Gx Sweat Patch in a broader range of conditions to include scenarios relevant to recreational exercisers. This study found significant correlations with the standard absorbent patch technique (Table 1) and good day-to-day reliability for LSR (9- 13%) and local sweat [Cl-] (11-14%). Furthermore, 148 subjects were added to the WBSR data set to expand the applicability of the predictive algorithm to include recreational exercise (fitness and running) and cooler environments (as low as 8°C).
The objective of the third study (Baker et al., 2022a) was to determine the ecological validity, as well as the criterion validity, of the Gx Sweat Patch and WBSR predictive algorithms by testing it in uncontrolled conditions with G-League basketball players during coach-led training sessions. This study corroborated the results of the first two papers, as we found significant correlations (Table 1) with the standard absorbent patch technique and extended the validity to elite basketball players, with or without tattooed skin, during moderate-intensity practice sessions that included a mix of non-contact drills and live high-contact scrimmages. Furthermore, there was no difference between measured and predicted WBSR (0.97 ± 0.41 L/h vs. 0.89 ± 0.35 L/h, p=0.22) and 95% Bland- Altman limits of agreement between methods was ±0.61 L/h. To put these results in practical context, whole-body sweat loss prediction error can be expressed as a percentage of the players’ body mass. Out of 49 subjects, n=35 (71%) predictions were within ±0.5% body mass. Forty-eight (98%) predictions were within ±1.0% body mass and n=1 (2%) was +1.5% of his body mass (Baker et al., 2022a). It is also relevant to note that in recent studies using only the absorbent patch technique, tattoos have had minimal to no effect on LSR, sweat [Na+], [Cl-], and [K+] during exercise (Beliveau et al., 2020; Keyes et al., 2022; Rogers et al., 2019). Thus, local sweat sampling and analyses from tattooed skin should have no impact on the practical interpretation of personalized sweat test results when conducted during exercise.
Finally, a fourth clinical trial (unpublished data) was conducted to expand the Gx data set to include outdoor cycling and broaden the age range (up to 65 years) and environmental conditions (up to 38°C). Once again, significant correlations with the absorbent patch data were achieved (Table 1).
Figure 3 shows the scatterplots of predicted versus measured whole-body sweat [Cl-] (r2=0.86) and the strong relation between whole-body sweat [Cl-] and sweat [Na+] (r2=0.98). Since Gx sweat [Cl-] alone accounts for 86% of the variation in whole-body sweat [Cl-], a simple linear regression model is used in the Gx App to generate sweat electrolyte results. Figure 4 shows the scatterplots of predicted versus measured WBSR for the final models developed from the four studies. Gx forearm sweating rate alone accounts for only ~55-65% of the variation in WBSR (Baker et al., 2020; 2022b); thus, a multiple regression model (incorporating additional factors) is used to predict WBSR. As shown in Figure 4, including body mass, sex, air temperature, sport, and exercise duration in the model increases the r2 to 66% (with a mean absolute percentage error (MAPE) of 21%), while also including energy expenditure increases the r2 further to 72% (with a MAPE of 19%). Table 2 shows the conditions and subject characteristics for which the Gx Sweat Patch and WBSR algorithms have been validated.
APPLYING SCIENCE-BASED HYDRATION STRATEGIES
Current guidelines regarding fluid replacement for the physically active recommend the prevention of modest levels of hypohydration (2% body mass deficit) while also avoiding overhydration (body mass gain) (McDermott et al., 2017; Sawka et al., 2007; Thomas et al., 2016). In addition, sodium should be ingested with fluid when sweat sodium losses are high, which may occur when there is some combination of moderate to prolonged duration exercise in the heat (>1–2 h), moderate-to-high sweating rates (>1.5 L/h), and/or moderate-to-high sweat sodium concentrations (>60 mmol/L) (Coyle, 2004; Shirreffs & Sawka, 2011; Thomas et al., 2016). When losses are high, some but not all, sweat sodium should be replaced during exercise. In a study where participants lost 2.8 g of sodium via sweating during 2 h cycling in the heat, replacement of ~50% of sodium losses led to better maintenance of plasma volume, stroke volume, and cycling performance than no sodium intake, but was not different from ~100% replacement (Hamouti et al., 2012).
Based on these guidelines a personalized fluid intake strategy can be developed from the sweat loss and electrolyte data obtained with the Gx Sweat Patch. For example, if there are two 70 kg individuals exercising for 90 min: Person A has a sweat loss of 2.3 L and a sweat sodium concentration of 70 mmol/L (3.6 g total sodium) while Person B has a sweat loss of 0.8 L and a sweat sodium concentration of 20 mmol/L (0.3 g total sodium). During the next 90 min workout of similar intensity and environmental conditions, Person A would be advised to drink ~1.0 L of fluid to prevent 2% hypohydration or up to 2.3 L of fluid if desired. In addition, during and/or after exercise, Person A would be advised to choose a fluid or food/gel/bar with sodium to mitigate an electrolyte imbalance and promote fluid retention. On the other hand, Person B would not need to take in any fluid to avoid 2% hypohydration during their 90 min workout. Person B could drink to thirst but would be advised to consume no more than 0.8 L in total and a small amount of sodium could be optional depending on personal preferences.
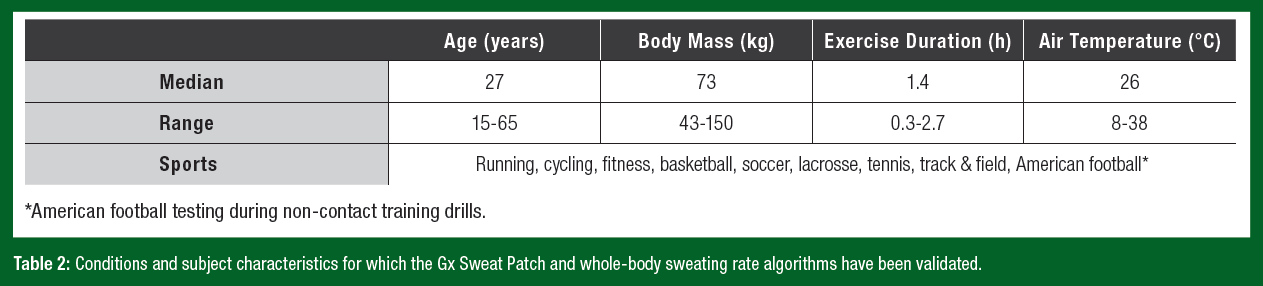
Gx DIGITAL APPLICATION
The Gx Smart Phone App is currently available on iOS and serves two main purposes as it relates to the Gx Sweat Patch: 1) image capture and algorithm processing pathway and 2) data visualization and practical recommendation generation via an intelligence engine.
Algorithms
Image processing algorithms are implemented on the Gx App to provide detection and analysis of LSR and local sweat [Cl-] over the full physiological range. Color swatches printed on the patch surface are included in the image processing to correct for color variations due to lighting conditions (e.g., color temperature). To enable users to capture images of sufficient quality and then quantify features of these images, custom software was developed using a combination of machine learning and traditional computer vision algorithms (Baker et al., 2022b).
The Gx App includes algorithms to predict WBSR and whole-body sweat [Na+] from the local sweat measurements. This is important since estimations of WBSR and electrolyte losses are required to inform hydration strategies. As described above, the algorithms were determined from simultaneous measurements of Gx patch and whole-body sweat data. A multiple regression model was derived to predict WBSR while participating in various team and individual sports under a range of environmental conditions. Inputs to the model included Gx sweating rate and various factors related to subject characteristics (body mass and sex), environment (air temperature), and exercise conditions (type of sport, energy expenditure, and exercise duration). A simple linear regression model was derived to predict whole-body sweat [Na+] from local sweat [Cl-] (Baker et al., 2020).
Intelligence Engine
As athletes are trending towards a more holistic understanding of athletic performance, exercise preparation and recovery, the intelligence engine within the Gx App combines with the Gx Sweat Patch and health and fitness applications (e.g., Apple Health) to provide insights and actionable recommendations to help athletes meet their goals. The Gx App enables recommendations focused on the athletic occasion (Thomas et al., 2016), which can be generated by the athlete through manual input or selected from one of nine different exercise programs contained within the Gx App. These pre-determined programs have been developed by a certified strength and conditioning specialist (CSCS) and have specific intentions (e.g., complete marathon training, core stability and conditioning, total body strength, etc.).
The intelligence engine assimilates information about the athlete and their exercise session to provide science-based hydration, fuel, and recovery strategies. Hydration recommendations are based on the individual sweat profiles determined from the Gx Sweat Patch and predictive algorithms as discussed above. Current guidelines suggest that macronutrient intake targets (Thomas et al., 2016) and potential supplement strategies (Maughan et al., 2018) can be developed from individual anthropometrics and the duration, intensity, and type of exercise performed. Thus, the Gx app requires the athlete to select the exercise type, duration, and the intended session rating of perceived exertion (s-RPE) for the workout to understand the training load and intensity (Foster, 1998). The intelligence engine applies the science-based guidelines to provide relevant macronutrient intake targets around the exercise occasion. For example, if an athlete inputs endurance exercise lasting more than one hour, the fuel target equivalent will be 30-60 g/h of carbohydrate. Similarly, a protein intake target for recovery nutrition can be determined for athletes looking to optimize muscle protein synthesis rates toward performance goals. For example, ~0.3 g/kg body mass of high-quality protein is recommended during the early recovery phase (0-2 h) or as soon as possible after the exercise session (Thomas et al., 2016).
Where appropriate, the supplements caffeine or creatine may be included in recommendations generated by the Gx App. Caffeine anhydrous consumed in a dose of 3-6 mg/kg body mass approximately 60 min before exercise has been shown to have ergogenic effects on endurance capacity and short-duration high-intensity exercise (Maughan et al., 2018; Peeling et al., 2018; Spriet, 2014). Recommendations from the intelligence engine are based on the lower end of the guideline (3 mg/ kg of body mass) to avoid potential side effects of higher doses. Lower amounts of caffeine (< 3mg/kg) combined with carbohydrate can be beneficial when provided before and during exercise (Maughan et al., 2018; Spriet, 2014). In terms of creatine supplementation, most of the documented performance benefits have been during high-intensity exercise bouts of < 30 sec, but it may also increase muscle strength, endurance, and lean body mass (Branch, 2003; Cooper et al., 2012; Lanhers et al., 2017; Maughan et al., 2018). Guidelines for creatine intake may include a loading phase consisting of 20 g/d for the first 5-7 days, followed by a maintenance phase of 3-5 g/d or possibly 0.03 g/ kg/d (Cooper et al., 2012; Hultman et al., 1996; Lanhers et al., 2017). Alternatively, sufficient muscle creatine levels can be attained with smaller doses (~3-5 g) over a longer period (~1 month) (Hultman et al., 1996). The latter approach is used as the basis for recommendations in the Gx App’s intelligence engine for potential creatine supplementation.
Gx App vs. Gx Teams
There are two types of Gx applications currently available on iOS. The Gx App is intended for use by individual athletes. It provides access to CSCS-designed exercise programs and macronutrient intake targets to better prepare and recover from exercise. The Gx App is fully customizable and synchronizes with other apps athletes might be using (e.g., Garmin, Strava, and Apple Health). It is also athlete specific (i.e., one phone, one athlete). On the other hand, Gx Teams is for sports practitioners. It allows practitioners to create teams to better track a roster of athletes as one phone and/or app can scan patches for multiple athletes (i.e., one phone, multiple athletes). Both apps utilize imaging processing algorithms to obtain results via the Gx Sweat Patch technology. The main difference between the apps lies in how the results are visualized. As the name suggests, Gx Teams allows the practitioner to monitor the unique sweat profiles across a group of athletes and enables real-time generation of personalized hydration recommendations for an entire team.
CONSIDERATIONS
While the Gx Patch is a valid and practical method for sweat testing in the field, it is not without limitations. The patch needs to maintain firm adhesion to the skin to provide valid results. In our research, the Gx Patch became unadhered from the skin in ~2-5% of subjects during no- or low-contact sports (Baker et al., 2020; 2022b) and ~14% of subjects during high-contact basketball scrimmaging (Baker et al., 2022a). By comparison, the standard absorbent patch fell off the forearm at a rate of ~0-1% (no or low contact) to 3% (high contact) during these same studies (Baker et al., 2020; 2022a,b). For adequate adhesion of the microfluidic patch to the skin, it is critical that the user follows the application instructions (detailed above) and avoids excessive physical contact with the patch. While the Gx patch has been used successfully during contact sports training (basketball and American football), delamination rates increase during live scrimmaging or gameplay.
It is also important to consider that there are minimum and, potentially, maximum wear times to obtain sweat profile results. The patch needs to be worn at least until orange fluid is visible in microchannel 1 to obtain sweating rate results and until purple fluid is visible in microchannel 2 to obtain sweat electrolyte results. Exact times vary depending upon the athlete’s sweating rate (see ‘Obtaining Sweat Profile Results’ for estimations). It is critical that an image of the patch is taken before the orange microchannel fills up, otherwise, the sweating rate data will be invalid. Note that if the microchannels do overflow purple or orange fluid may go onto the skin. The food dyes are harmless and can be washed off with soap and water.
CONCLUSIONS
A series of clinical trials were conducted to compare results from the wearable microfluidic Gx Sweat Patch and App-based algorithms with standard techniques for sweat testing. The main findings were:
- LSR and local sweat [Cl−] data from the Gx Sweat Patch were significantly correlated with those of the standard absorbent patch technique (r=0.77-0.93).
- The test-retest (day-to-day) reliability of the Gx Sweat Patch was similar to the reference techniques for both LSR (9-13%) and local sweat [Cl−] (11-14%).
- Robust predictive algorithms were developed to determine WBSR (r2=0.72, MAPE=19%) and whole body sweat [Cl-] (r2=0.86, MAPE=13%) from the Gx Sweat Patch data and other inputs related to the individual and their workout.
- There is a strong relation between whole-body sweat [Cl-] and whole-body sweat [Na+] (r2=0.98), thus enabling accurate prediction of sweat [Na+] using the Gx Sweat Patch and App.
In conclusion, the Gx platform is a validated tool that improves the accessibility of sweat analytics in real-world settings, with applications in competitive athletics and recreational exercise in a wide range of conditions.
PRACTICAL APPLICATIONS
- The Gx Sweat Patch should be applied to the left ventral forearm before exercise. To obtain sweat profile data the athlete or practitioner should use the Gx App to take an image of the Gx Sweat Patch while it is still on the skin. The image should be taken after completion of the exercise session or just before the orange microchannel fills up during exercise, whichever comes first.
- The minimum wear time to obtain sweat profile results (i.e., until colored sweat is visible) varies from ~20 min for high sweat rates to 60 min for low sweat rates. For very heavy sweaters the maximum wear time before the microchannels fill up could be around 60 min or less.
- The Gx Sweat Patch can be worn on tattooed skin and during a variety of activities and environmental conditions. However, there is a higher risk of patch delamination when worn during high-contact sports (e.g., basketball games).
- The Gx App generates personalized fluid intake recommendations based on the user’s sweat profile. The user should then follow the recommendations to properly rehydrate after the workout and/or during their next workout of similar intensity, duration, and environmental conditions.
Authors Lindsay Baker and Anthony Wolfe are employed by the Gatorade Sports Science Institute, a division of PepsiCo, Inc. The views expressed in this article are those of the authors and do not necessarily reflect the position or policy of PepsiCo, Inc.
REFERENCES
Baker, L.B. (2017). Sweating rate and sweat sodium concentration in athletes: A review of methodology and intra/interindividual variability. Sports Med, 47(Suppl 1):111-128.
Baker, L.B., C.T. Ungaro, B.C. Sopena, R.P. Nuccio, A.J. Reimel, J.M. Carter, J.R. Stofan, and K.A. Barnes (2018). Body map of regional versus whole body sweating rate and sweat electrolyte Concentrations in Men and Women during Moderate Exercise-Heat Stress. J. Appl. Physiol. 124:1304-1318.
Baker, L.B., P.J.D. De Chavez, C.T. Ungaro, B.C. Sopena, R.P. Nuccio, A.J. Reimel, and L.A. Barnes (2019). Exercise intensity effects on total sweat electrolyte losses and regional vs. whole-body sweat [Na(+)], [Cl(-)], and [K(+)]. Eur. J. Appl. Physiol. 119:361-375.
Baker, L.B., J.B. Model, K.A, Barnes, M.L. Anderson, S.P. Lee, K.A. Lee, S.D. Brown, A.J. Reimel, T.J. Roberts, R.P. Nuccio, J.L. Bonsignore, C.T. Ungaro, J.M. Carter, W. Li, M.S. Seib, J.T. Reeder, A.J. Aranyosi, J.A. Rogers, and R. Ghaffari (2020). Skin-interfaced microfluidic system with personalized sweating rate and sweat chloride analytics for sports science applications. Sci. Adv. 6:eabe3929.
Baker, L.B., M.A. King, D.M. Keyes, S.D. Brown, M.D. Engel, M.S. Seib, A.J. Aranyosi, and R. Ghaffari (2022a). Sweating rate and sweat chloride concentration of elite male basketball players measured with a wearable microfluidic device versus the standard absorbent patch method. Int. J. Sport Nutr. Exerc. Metab. 32:342-349.
Baker, L.B., M.S. Seib, K.A. Barnes, S.D. Brown, M.A. King, P.J.D. De Chavez, S. Qu, J. Archer, A.S. Wolfe, J.R. Stofan, J.M. Carter, D.E. Wright, J. Wallace, D. Yang, S. Liu, J. Anderson, T. Fort, W. Li, J.A. Wright, . . . R. Ghaffari (2022b). Skin-interfaced microfluidic system with machine learning-enabled image processing of sweat biomarkers in remote settings. Adv. Materials Tech. 7(11) https://doi.org/ https://doi. org/10.1002/admt.202200249
Barnes, K.A., M.L. Anderson, J.R. Stofan, K.J. Dalrymple, A.J. Reimel, T.J. Roberts, R.K. Randell, C.T. Ungaro, and L.B. Baker (2019). Normative data for sweating rate, sweat sodium concentration, and sweat sodium loss in athletes: An update and analysis by sport. J. Sports Sci. 37:2356-2366.
Beliveau, J., M. Perreault-Briere, D. Jeker, T.A. Deshayes, A. Duran-Suarez, L.B. Baker, and E.D.B. Goulet (2020). Permanent tattooing has no impact on local sweat rate, sweat sodium concentration and skin temperature or prediction of whole-body sweat sodium concentration during moderate-intensity cycling in a warm environment. Eur J Appl Physiol. 120:1111-1122.
Belval, L.N., Y. Hosokawa, D.J. Casa, W.M. Adams, L.E. Armstrong, L.B. Baker, L. Burke, S. Cheuvront, G. Chiampas, J. Gonzalez-Alonso, R.A. Huggins, S.A. Kavouras, E.C. Lee, B.P. McDermott, K. Miller, Z. Schlader, S. Sims, R.L. Stearns, C. Troyanos, and J. Wingo (2019). Practical hydration solutions for sports. Nutrients 11:1550.
Branch, J.D. (2003). Effect of creatine supplementation on body composition and performance: a meta-analysis. Int. J. Sport Nutr. Exerc. Metab. 13:198-226.
Cheuvront, S.N., and R.W. Kenefick (2017). CORP: Improving the status quo for measuring whole body sweat losses (WBSL). J. Appl. Physiol. 123:632-636.
Cooper, R., F. Naclerio, J. Allgrove, and A. Jimenez (2012). Creatine supplementation with specific view to exercise/sports performance: an update. J. Int. Soc. Sports Nutr. 9:33.
Coyle, E.F. (2004). Fluid and fuel intake during exercise. J. Sports Sci. 22:39-55.
Dziedzic, C.E., M.L. Ross, G.J. Slater, and L.M. Burke (2014). Variability of measurements of sweat sodium using the regional absorbent patch method. Int. J. Sports Physiol. Perform. 5:832-838.
Foster, C. (1998). Monitoring training in athletes with reference to overtraining syndrome. Med. Sci. Sports Exerc. 30:1164-1168.
Hamouti, N., V.E. Fernandez-Elias, J.F. Ortega, and R. Mora-Rodriguez (2012). Ingestion of sodium plus water improves cardiovascular function and performance during dehydrating cycling in the heat. Scand. J. Med. Sci. Sports. 24:507-518.
Hew-Butler, T., M.H. Rosner, S. Fowkes-Godek, J.P. Dugas, M.D. Hoffman, D.P. Lewis, R.J. Maughan, K.C. Miller, S.J. Montain, N.J. Rehrer, W.O. Roberts, I.R. Rogers, A.J. Siegel, K.J. Stuempfle, J.M. Winger, and J.G. Verbalis (2015). Statement of the 3rd international exercise-associated hyponatremia consensus development conference. Br. J. Sports Med. 49:1432-1446.
Hultman, E., K. Soderlund, J.A. Timmons, G. Cederblad, and P.L. Greenhaff (1996). Muscle creatine loading in men. J. Appl. Physiol. 81:232-237.
Keyes, D.M., S.D. Brown, M.A. King, M.D. Engel, M. Ciciora-Gold, P.J.D. Chavez, and L.B. Baker (2022). Multiple regression analyses to determine the effect of sweating rate and tattoo characteristics on sweat outcome measures during exercise. Eur. J. Appl. Physiol. 122:2163-2174.
Lanhers, C., B. Pereira, G. Naughton, M. Trousselard, F.X. Lesage, and F. Dutheil (2017). Creatine supplementation and upper limb strength performance: a systematic review and meta-analysis. Sports Med. 47:163-173.
Lemon, P.W., K.E. Yarasheski, and D.G. Dolny (1986). Validity/reliability of sweat analysis by whole-body washdown vs. regional collections. J. Appl. Physiol. 61:1967-1971.
Maughan, R.J., S.J. Merson, N.P. Broad, and S.M. Shirreffs (2004). Fluid and electrolyte intake and loss in elite soccer players during training. Int. J. Sport Nutr. Exerc. Metab. 14:333-346.
Maughan, R.J., L.M. Burke, J. Dvorak, D.E. Larson-Meyer, P. Peeling, S.M. Phillips, E.S. Rawson, N.P. Walsh, I. Garthe, H. Geyer, R. Meeusen, L.J.C. van Loon, S.M. Shirreffs, L.L. Spriet, M. Stuart, A. Vernec, K. Currell, V.M. Ali, R.G. Budgett, A. Ljungqvist, M. Mountjoy, Y.P. Pitsiladis, T. Soligard and L. Engebretsen, L. (2018). IOC consensus statement: dietary supplements and the high-performance athlete. Br. J. Sports Med. 52:439-455.
McDermott, B.P., S.A. Anderson, L.E. Armstrong, D.J. Casa, S.N. Cheuvront, L. Cooper, W.L. Kenney, F.G. O'Connor, and W.O. Roberts (2017). National Athletic Trainers' Association position statement: Fluid replacement for the physically active. J. Athl. Train. 52:877- 895.
Morris, N.B., M.N. Cramer, S.G. Hodder, G. Havenith, and O. Jay (2013). A comparison between the technical absorbent and ventilated capsule methods for measuring local sweat rate. J. Appl. Physiol. 114:816-823.
Peeling, P., M.J. Binnie, P.S.R. Goods, M. Sim, and L.M. Burke (2018). Evidence-based supplements for the enhancement of athletic performance. Int. J. Sport Nutr. Exerc. Metab. 28:178-187.
Rogers, E., C. Irwin, D. McCartney, G.R. Cox, and B. Desbrow (2019). Tattoos do not affect exercise-induced localised sweat rate or sodium concentration. J. Sci. Med Sport. 22:1249-1253.
Sawka, M.N., L.M. Burke, E.R. Eichner, R.J. Maughan, S.J. Montain, and N.S. Stachenfeld (2007). American College of Sports Medicine position stand. Exercise and fluid replacement. Med. Sci. Sports Exerc. 39:377-390.
Shirreffs, S.M., and R.J. Maughan (1997). Whole body sweat collection in humans: an improved method with preliminary data on electrolyte content. J. Appl. Physiol. 82:336-341.
Shirreffs, S.M., and M.N. Sawka (2011). Fluid and electrolyte needs for training, competition, and recovery. J. Sports Sci. 29(Suppl 1):S39-S46.
Spriet, L.L. (2014). Exercise and sport performance with low doses of caffeine. Sports Med; 44(Suppl 2): S175-S184.
Thomas, D.T., K.A. Erdman, and L.M. Burke (2016). American College of Sports Medicine joint position statement. Nutrition and athletic performance. Med. Sci. Sports Exerc. 48:543-568.
Verde, T., R.J. Shephard, P. Corey, and R. Moore (1982). Sweat composition in exercise and in heat. J. Appl. Physiol. 53:1540-1545.