KEY POINTS
-
Polyphenols are produced by plants and fulfill a number of functions including pathogen and antioxidant defense. They contribute to the taste and color characteristics of fruit and vegetables, but ~90% of dietary polyphenols escape absorption in the small intestine and are subsequently made bioavailable by the actions of the gut bacteria in the colon.
-
Polyphenol supplementation produces antioxidant effects via inhibition of superoxide-generating enzymes such as nicotinamide adenine dinucleotide phosphate (NADPH) oxidase and increased synthesis of endogenous antioxidant enzymes via the antioxidant response element pathway. They also exert anti-inflammatory effects via inhibition of the cyclooxygenase enzymes that produce pro-inflammatory prostaglandins.
-
Consumption of ~300 mg polyphenols within the hour prior to exercise appears to enhance performance of endurance and repeated sprint exercise in recreationally active participants, mediated via vascular mechanisms. However, only a small number of studies have been conducted to date and more research is needed to corroborate these findings, to determine the influence of training status, and to optimize the supplementation protocol.
-
Recovery of muscle strength and exercise performance after intensive exercise is enhanced by polyphenol supplementation, most likely due to protection against oxidative damage. However, our understanding of the mechanisms is currently based on measurements of indirect plasma biomarkers rather than analysis of the damaged muscle itself.
-
The optimal dose and blend of polyphenols to support recovery from muscle damage is not currently known, but consumption of ~1200 mg/ day Montmorency cherry or pomegranate polyphenols for three or more days prior to exercise appears to be consistently effective in enhancing recovery.
INTRODUCTION
Fruits, vegetables and fruit-derived polyphenol supplements contain a blend of polyphenols; thus, the pharmacokinetics and metabolism after ingestion of whole foods or fruit-derived supplements are highly complex. Consequently, there are still many unanswered questions relating to the optimal blend and dose of polyphenols to enhance exercise performance and recovery. The taste and color characteristics of fruits and vegetables are strongly influenced by the polyphenol content. Polyphenols are ubiquitous within plants, where they are involved in a diverse range of critical processes including growth, pigmentation, pollination, resistance to pathogens and environmental stressors (Duthie et al., 2003). The polyphenol content and blend of plants are determined by the plant species, growing conditions (sunlight, water, nutrient availability, temperature), post-harvest processing, transport, and storage conditions (Oracz et al., 2015). Polyphenols have a common chemical structure with two or more hydroxyl groups attached to one or more benzene rings and can be classified into four main families: lignans, phenolic acids, stilbenes and flavonoids. Of these, flavonoids are the most abundant. Table 1 provides a summary of example compounds and key dietary sources of the different polyphenol families. There is considerable variability in the polyphenol content of foods on the supermarket shelves, and in the many polyphenol-rich fruit-derived supplements that are now commercially available.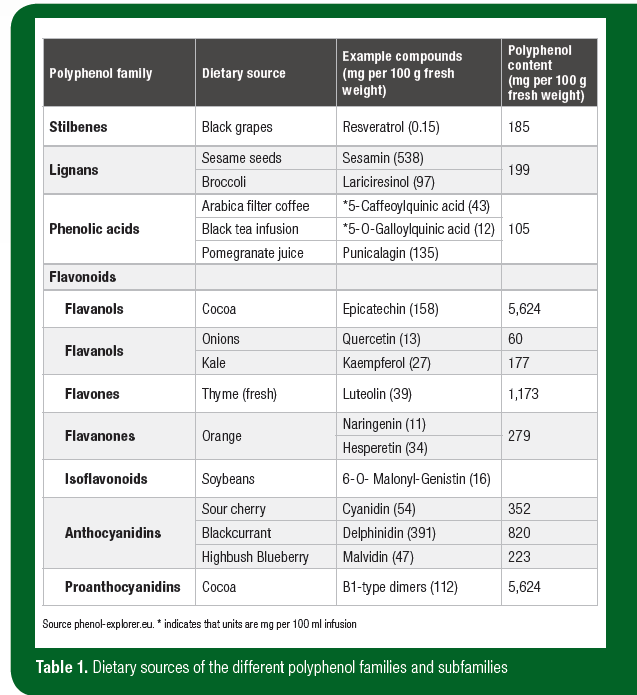
The absorption and metabolism of polyphenols is very complex as there are many thousands of polyphenol compounds present within plants which interact with one another, forming a large number of different metabolites upon absorption (Crozier et al., 2010). Approximately 90% of polyphenols pass undigested through the small intestine to the colon, where they are acted upon by enzymes present in the bacteria, producing metabolites such as phenolic acids, which can then be absorbed. Ingestion of a single polyphenol can give rise to many different metabolites. For example, in the 48 h following ingestion of 13C-labelled cyanidin-3-glucoside, 17 different labelled metabolites were detected in the plasma (de Ferrars et al., 2014).
Polyphenol Properties
Polyphenols possess radical scavenging properties related to their chemical structure. However, maximal plasma concentrations of polyphenols and their metabolites are relatively low (0.1– 22 μM) when compared to the concentration of plasma urate (150– 450 μM) which is also an important antioxidant in the plasma compartment. It seems unlikely, therefore, that plasma phenolics are effective direct antioxidants in vivo. There is now growing evidence that phenolics increase endogenous antioxidant capacity through signalling via the nuclear factor-like 2 (Nrf2)/antioxidant response element (ARE) pathway, resulting in increased synthesis of downstream endogenous antioxidants such as superoxide dismutase, catalase and peroxiredoxin (Huang et al., 2015). Paradoxically, it seems that antioxidant effects of polyphenols arise from their pro-oxidant action after in vivo exposure to reactive oxygen species (ROS). Polyphenols also possess anti-inflammatory properties and have been shown in vitro to reduce signalling via the pro-inflammatory nuclear factor kappa-light-chain-enhancer of activated B cells (NF-κB) pathway and to inhibit cyclooxygenase enzymes, COX-1 and COX-2 (Esposito et al., 2014), which are also the target of nonsteroidal anti-inflammatory drugs.
Consumption of fruit-derived polyphenols, including those from cherries, blueberries, blackcurrant, pomegranate, and cocoa, lowers plasma markers of oxidative damage and inflammation. Acute and chronic supplementation with polyphenols also improves vascular function, specifically nitric oxide (NO) dependent flow mediated dilatation (FMD). A meta-analysis revealed that acute supplementation with a mixture of flavonoids increased FMD by 2.33% (based on 18 studies) and by 0.73% with chronic supplementation (based on 14 studies) (Kay et al., 2012). The optimal dose identified was 500 mg/day total flavonoids or 300 mg/day of procyanidins. The increase in NO bioavailability is likely achieved through a variety of mechanisms acting synergistically to 1) activate endothelial nitric oxide synthase (Chalopin et al., 2010), 2) inhibit superoxide-producing enzymes such as NADPH oxidase (Maraldi, 2013), thus reducing depletion of NO due to peroxynitrite formation from NO and superoxide, and 3) signal through Nrf2 and increase endogenous antioxidant capacity (Ramirez-Sanchez et al., 2013).
There is thus a strong rationale to suggest that polyphenols may improve exercise performance and recovery from intensive exercise due to their antioxidant, anti-inflammatory and vasoactive properties (Bowtell & Kelly, 2019). The goal of this Sports Science Exchange article is to provide a brief summary of the current research evidence in this area.
POLYPHENOL SUPPLEMENTATION FOR EXERCISE PERFORMANCE
Skeletal muscle is a net producer of reactive oxygen species from a variety of sources including the mitochondrial respiratory chain and enzymatic sources such as NADPH oxidase and xanthine oxidase. ROS generation increases during exercise in an intensity-dependent fashion and can exceed muscle antioxidant capacity resulting in disturbed redox balance during high intensity or prolonged exercise (Bailey et al., 2007). This disturbance to redox balance is implicated in fatigue development via impaired blood flow and vasodilatory capacity, altered calcium handling and sensitivity, and development of central fatigue (Figure 1, for review see Powers & Jackson, 2008). Therefore, it is plausible that antioxidant supplementation may counteract fatigue and enhance performance, via enhanced perfusion of the exercising muscle, as well as better maintained excitation-contraction coupling and central drive.
Acute Supplementation
Only a handful of studies have investigated whether acute polyphenol supplementation (single dose < 3 h pre-exercise) affects exercise performance. Timing of consumption appears to be an important factor as ergogenic effects were only observed in those studies where polyphenols were consumed within 1 h of exercise. This is likely to coincide with the peak concentration of the plasma phenolic metabolites and, therefore, maximal physiological effects. When consumed 30 min prior to exercise, pomegranate (1000 mg pomegranate extract) (Roelofs et al., 2017; Trexler et al., 2014) and ecklonia cava extract (72 mg polyphenols) (Oh et al., 2010) enhanced treadmill time to exhaustion, and peak and average power during repeated cycle sprints in recreationally active participants. Polyphenol combinations derived from green tea, grape and pomegranate, or grape and apple (500 mg polyphenols) consumed 1 h prior to exercise increased peak and average power during repeated cycle sprints (Cases et al., 2017) or increased time to exhaustion during a maximal incremental treadmill running test, and cycling at 70% maximum aerobic power (Deley et al., 2017) in recreationally active participants. However, pomegranate extract or cocoa flavanols consumed 1.5 h or longer prior to exercise did not produce ergogenic effects (Decroix et al., 2017; Crum et al., 2017; Roelofs et al., 2017; Trexler et al., 2014). These studies involved trained athletes, so it is not clear whether training status may also influence efficacy; although, trained cyclists experienced modest improvements in end sprint performance 1.5 h after ingestion of Montmorency cherry concentrate (Keane et al., 2018).
The observed ergogenic effects in recreationally active subjects are likely to involve vascular mechanisms, with lowered pulse pressure, and increased brachial artery diameter and flow observed in conjunction with performance improvements (Cases et al., 2017; Roelofs et al., 2017; Trexler et al., 2014).
Chronic Supplementation
Supplementation with blackcurrant powder (300 mg/day with 105 mg/ day anthocyanins) for 7 days enhanced 16.1 km cycling time trial performance (Cook et al., 2015) and high-intensity intermittent running distance to exhaustion (Perkins et al., 2015), reduced fatigue index with repeated sprints in recreationally active participants (Godwin et al., 2017; Willems et al., 2016), and induced a small (0.8%) improvement in repeated 4 km cycling time trial performance in trained individuals (Murphy et al., 2017). Braakhuis et al. (2014) also found a possible improvement in 5 km time trial performance for faster female runners after 3 wk of blackcurrant polyphenol supplementation (300 mg/day anthocyanins) during a period of intensified training, with evidence of a performance decrement after vitamin C supplementation in this randomized crossover trial. Cherry powder supplementation for 7 days enhanced 15 km time trial performance in trained male cyclists (Morgan et al., 2019). However, findings were not consistent, as 7 days of pomegranate (1800 mg polyphenols) supplementation (Trinity et al., 2014), and 2 wk of dark chocolate (108 mg catechins and 88 mg flavanols) supplementation (Allgrove et al., 2011) did not enhance performance.
From the available evidence, chronic polyphenol consumption seems to produce ergogenic effects for recreationally active participants and, to a lesser extent, for trained athletes. The most likely mechanisms appear to be reduced exposure to, or increased capacity to, detoxify ROS and reactive nitrogen species (RNS) or increased antioxidant enzyme activity. These responses seem to occur in parallel with enhanced vascular function, possibly resulting in improved muscle perfusion and enhanced oxygen extraction (Richards et al., 2010).
POLYPHENOL SUPPLEMENTATION FOR ENHANCED RECOVERY
Exercise-induced muscle damage involves both mechanical and biochemical processes. The initial damage, induced by exposure to high mechanical forces and increased ROS exposure, triggers a potent inflammatory response with damaged fibers releasing pro-inflammatory cytokines, which serve as chemo-attractants for neutrophils and macrophages and activate ROS-generating enzymes within the muscle (for review see Peake et al., 2017). Neutrophil infiltration and activation occur within 2 h of damage, which releases ROS and proteolytic enzymes that may exacerbate the initial muscle damage but also facilitates regeneration by removal of debris and activation of satellite cells. Shortly after neutrophil infiltration, macrophages derived from blood monocytes accumulate within the damaged tissue. Their role is to scavenge debris and apoptotic cells and, in addition, release a range of growth factors and other substances that trigger remodeling of extracellular matrix, and contractile and vascular elements. The central involvement of ROS generation and inflammation within the muscle damage and healing process suggests that there is a clear rationale for supplementation with polyphenols to enhance recovery (Figure 2).
Fruit-derived polyphenol (Montmorency cherry (MC), pomegranate and blueberry) supplementation enhances restoration of muscle function and reduces soreness after intensive exercise. MC supplementation has been shown to enhance recovery of muscle function or exercise performance after intensive exercise in five of nine published studies investigating this question (Bell et al., 2015, 2016; Bowtell et al., 2011; Connolly et al., 2006; Howatson et al., 2010). These studies involved trained athletes as well as recreationally active individuals, so unlike the acute performance effects of polyphenol supplementation, it seems that beneficial effects on recovery are accessible to both trained and less well-trained individuals. The efficacy of the supplement was not influenced by the mode of exercise used to induce muscle damage, since different approaches or muscle groups were damaged in each of these studies. MC polyphenols were provided in the form of a juice drink, consumed morning and evening for at least 3 days prior to exercise and provided at least 1200 mg polyphenols/day in the studies where enhanced recovery was evident. In the studies that reported no effect on enhanced recovery of muscle function or exercise performance, either a lower (and presumably insufficient) dose of MC was provided in powder form (Beals et al., 2017; Levers et al., 2015), or the intensive exercise task did not induce a measurable decline in muscle strength (McCormick et al., 2016) or exercise performance (Bell et al., 2014), thus by definition making it impossible to improve recovery.
Pomegranate juice consumption has also been shown to enhance recovery of elbow flexor (Machin et al., 2012; Trombold et al., 2010, 2011) and knee extensor (Machin et al., 2012) muscle function after intensive exercise in male subjects. However, Trombold et al. (2011) reported that the knee extensors in resistance-trained men were relatively refractory to muscle damage (15-20% reduction in isometric strength vs. 25-35% loss of elbow flexor isometric strength), which may have contributed to the lack of pomegranate polyphenol effects in the knee extensors of this group. However, in recreationally active men, the recovery of both elbow flexor and knee extensor strength was enhanced by consumption of pomegranate concentrate providing 650 mg polyphenols either once or twice/day for 3 days prior to exercise (Machin et al., 2012; Trombold et al., 2010), suggesting that this lower polyphenol dose was equally effective in this population. It should be mentioned that the Machin et al. (2012) study was only reported as an abstract. Blueberry supplementation consumed in the form of a smoothie on the day of exercise (1,360 mg polyphenols) and during 2 days recovery (420 mg polyphenols/day) enhanced recovery of knee extensor strength after unilateral eccentric exercise in recreationally active women (McLeay et al., 2012).
The effects of cherry polyphenol supplementation on muscle soreness after intensive exercise are far less consistent, with MC supplementation reducing muscle soreness after intensive exercise in half of the published studies (Bell et al., 2016; Connolly et al., 2006; Kuehl et al., 2010; Levers et al., 2015), but not in the other published studies in which soreness was reported (Beals et al., 2017; Bowtell et al., 2011; Howatson et al., 2010; McCormick et al., 2016). Pomegranate supplementation reduced muscle soreness after eccentric elbow flexor exercise in resistance-trained men (Trombold et al., 2011), but not in the other muscles or studies, and blueberry supplementation did not reduce muscle soreness (McLeay et al., 2012). There was no clear pattern to explain this variation, but studies were not consistent in terms of dietary control as some ensured that participants refrained from polyphenol consumption before the study commenced and some did not. In three studies, favorable effects on recovery of both muscle strength and soreness were evident; in others, favorable effects on muscle function but not soreness were observed. By definition, soreness is a highly subjective measure even when pressure pain tolerance is measured using an algometer, and although important for athlete performance, it is difficult to reliably and objectively quantify. The quantification of inflammation within the damaged muscle itself, alongside measures of muscle soreness, could be an important step forward in better understanding the effects of polyphenols.
PRACTICAL APPLICATIONS
- There is preliminary evidence to suggest that consumption of ~300 mg polyphenols in the hour prior to exercise may enhance endurance and repeated sprint performance, most likely due to improved muscle perfusion.
- Optimization of recovery post-exercise is imperative during heavy training and competition schedules where limited time for recovery is available. Current evidence suggests that supplementation with > 1000 mg polyphenols/day for 3 or more days prior to and following exercise will enhance recovery following sporting events that induce muscle damage.
- This dose of polyphenols could be provided by consuming ~450 g blueberries, 120 g blackcurrants or 300 g Montmorency cherries (see Table 1 and phenolexplorer.eu). However, the polyphenol content of fruits varies considerably depending upon the plant species, growing conditions and post-harvest processing.
- Practitioners should consider their athletes’ personal taste preferences and lifestyle demands when recommending individual supplementation protocols to ensure compliance, and whole-fruit polyphenol supplements may provide a more convenient and consistent polyphenol source.
SUMMARY
In summary, the consumption of MC juice or concentrate providing 600 mg polyphenols morning and evening for at least 3 days prior to exercise and during recovery has consistently been shown to improve recovery of muscle function. Protection of the contracted muscle from oxidative damage and suppression of inflammation are likely to be the mechanisms that underpin these favorable effects. Consumption of ~300 mg fruit-derived polyphenols in the hour prior to exercise appears to enhance exercise performance, most likely via enhanced muscle perfusion. Further studies are required to identify the optimal dose, frequency and duration of consumption, and to improve our understanding of the mechanisms of action.
REFERENCES
Allgrove, J., E. Farrell, M. Gleeson, G. Williamson, and K. Cooper (2011). Regular dark chocolate consumption's reduction of oxidative stress and increase of free-fatty-acid mobilization in response to prolonged cycling. Int. J. Sport Nutr. Exerc. Met. 21:113-123.
Bailey, D.M., L. Lawrenson, J. McEneny, I.S. Young, P.E. James, S.K. Jackson, R.R. Henry, O. Mathieu-Costello, J.M. McCord, and R.S. Richardson (2007). Electron paramagnetic spectroscopic evidence of exercise-induced free radical accumulation in human skeletal muscle. Free Radical Res. 41:182-190.
Beals, K., K.F. Allison, M. Darnell, M. Lovalekar, R. Baker, D.C. Nieman, et al. (2017). The effects of a tart cherry beverage on reducing exercise-induced muscle soreness. Isokin. Exerc. Sci. 25:53-63.
Bell, P.G., I.H. Walshe, G.W. Davison, E. Stevenson, and G. Howatson (2014). Montmorency cherries reduce the oxidative stress and inflammatory responses to repeated days high-intensity stochastic cycling. Nutrients 6:829-843.
Bell, P.G,, I.H. Walshe, G.W. Davison, E.J. Stevenson, and G. Howatson (2015). Recovery facilitation with Montmorency cherries following high-intensity, metabolically challenging exercise. Appl. Physiol, Nutr. Met. 40:414-423.
Bell, P.G., E. Stevenson, G.W. Davison, and G. Howatson (2016). The effects of Montmorency tart cherry concentrate supplementation on recovery following prolonged, intermittent exercise. Nutrients 8(7):E441.
Bowtell, J.L., D.P. Sumners, A. Dyer, P. Fox, and K.N. Mileva (2011). Montmorency cherry juice reduces muscle damage caused by intensive strength exercise. Med. Sci. Sports Exerc. 43:1544-1551.
Bowtell, J.L. and V. Kelly (2019). Fruit-derived polyphenol supplementation for athlete recovery and
performance. Sports Med. 2019.
Braakhuis, A.J., W.G. Hopkins, and T.E. Lowe (2014). Effects of dietary antioxidants on training and performance in female runners. Eur. J. Sport Sci. 14:160-168.
Cases, J., C. Romain, C. Marin-Pagan, L.H. Chung, J.M. Rubio-Perez, C. Laurent, S. Gaillet, E. Prost-Camus, M. Prost, and P.E. Alcaraz (2017). Supplementation with a polyphenol-rich extract, perfload (r), improves physical performance during high-intensity exercise: a randomized, double blind, crossover trial. Nutrients 9(4):E421.
Chalopin, M., A. Tesse, M.C. Martinez, D. Rognan, J.F. Arnal, and R. Andriantsitohaina (2010). Estrogen receptor alpha as a key target of red wine polyphenols action on the endothelium. PLos One 5(1):e8554.
Connolly, D.A., M.P. McHugh, and O.I. Padilla-Zakour (2006). Efficacy of a tart cherry juice blend in preventing the symptoms of muscle damage. Br. J. Sports Med. 40:679-683.
Cook, M.D., S.D. Myers, S.D. Blacker, and M.E. Willems (2015). New Zealand blackcurrant extract improves cycling performance and fat oxidation in cyclists. Eur. J. Appl. Physiol. 115:2357- 2365.
Crozier, A., D. Del Rio, and M.N. Clifford (2010). Bioavailability of dietary flavonoids and phenolic compounds. Mol. Asp. Med. 31:446-467.
Crum, E.M., A.M. Che Muhamed, M. Barnes, and S.R. Stannard (2017). The effect of acute pomegranate extract supplementation on oxygen uptake in highly-trained cyclists during high-intensity exercise in a high altitude environment. J. Int. Soc. Sports Nutr. 14:14.
de Ferrars, R.M., C. Czank, Q. Zhang, N.P. Botting, P.A. Kroon, A. Cassidy, and C.D. Kay (2014). The pharmacokinetics of anthocyanins and their metabolites in humans. Br. J. Pharmacol. 171:3268-3282.
Decroix, L., C. Tonoli, D.D. Soares, A. Descat, M.J. Drittij-Reijnders, A.R. Weseler, A. Bast, W. Stahl, E. Heyman, and R. Meeusen (2017). Acute cocoa Flavanols intake has minimal effects on exercise-induced oxidative stress and nitric oxide production in healthy cyclists: a randomized controlled trial. J. Int. Soc. Sports Nutr. 14:28.
Deley, G., D. Guillemet, F.A. Allaert, and N. Babault (2017). An acute dose of specific grape and apple polyphenols improves endurance performance: a randomized, crossover, double-blind versus placebo controlled study. Nutrients 9(8):E917.
Duthie, G.G., P.T. Gardner, and J.A. Kyle (2003). Plant polyphenols: are they the new magic bullet? Proc. Nutr. Soc. 62:599-603.
Esposito, D., A. Chen, M.H. Grace, S. Komarnytsky, and M.A. Lila (2014). Inhibitory effects of wild blueberry anthocyanins and other flavonoids on biomarkers of acute and chronic inflammation in vitro. J, Agric. Food Chem. 62:7022-7028.
Godwin, C., M.D. Cook, and M.E.T. Willems (2017). Effect of New Zealand blackcurrant extract on performance during the running based anaerobic sprint test in trained youth and recreationally active male football players. Sports 5(3):E69.
Howatson, G., M.P. McHugh, J.A. Hill, J. Brouner, A.P. Jewell, K.A. van Someren, R.E. Shave, and S.A. Howatson (2010). Influence of tart cherry juice on indices of recovery following marathon running. Scand. J. Med. Sci. Sports 20:843-852.
Huang, Y., W. Li, Z.Y. Su, and A.N. Kong (2015). The complexity of the Nrf2 pathway: beyond the antioxidant response. J. Nutr. Biochem. 26:1401-1413.
Kay, C.D., L. Hooper, P.A. Kroon, E.B. Rimm, and A. Cassidy (2012). Relative impact of flavonoid composition, dose and structure on vascular function: A systematic review of randomised controlled trials of flavonoid-rich food products. Mol. Nutr. Food Res. 56:1605-1616.
Keane, K.M., S.J. Bailey, A. Vanhatalo, A.M. Jones, and G. Howatson (2018). Effects of montmorency tart cherry (L-Prunus Cerasus) consumption on nitric oxide biomarkers and exercise performance. Scand. J. Med. Sci. Sports 28:1746-1756.
Kuehl, K.S., E.T. Perrier, D.L. Elliot, and J.C. Chesnutt (2010). Efficacy of tart cherry juice in reducing muscle pain during running: a randomized controlled trial. J. Int. Soc. Sports Nutr. 7:17.
Levers, K., R. Dalton, E. Galvan, C. Goodenough, A. O'Connor, S. Simbo, N. Barringer, S.U. Mertens-Talcott, C. Rasmussen, M. Greenwood, S. Riechman, S. Crouse, and R.B. Kreider (2015). Effects of powdered Montmorency tart cherry supplementation on an acute bout of intense lower body strength exercise in resistance trained males. J. Int. Soc. Sports Nutr. 12:41.
Machin, D.R., K.M. Christmas, T.H. Chou, S.C. Hill, D. Van Pelt, J.R. Trombold, and E.F. Coyle (2012). Dose response effects of pomegranate juice concentrate supplementation on DOMS. Med. Sci. Sports Exerc. 44:315 (abstract).
Maraldi, T. (2013). Natural compounds as modulators of NADPH oxidases. Oxid. Med. Cell. Longev. 13:271602.
McCormick, R., P. Peeling, M. Binnie, B. Dawson, and M. Sim (2016). Effect of tart cherry juice on recovery and next day performance in well-trained Water Polo players. J. Int. Soc. Sports Nutr. 13:41.
McLeay, Y., M.J. Barnes, T. Mundel, S.M. Hurst, R.D. Hurst, and S.R. Stannard (2012). Effect of New Zealand blueberry consumption on recovery from eccentric exercise-induced muscle damage. J. Int. Soc. Sports Nutr. 9(1):19.
Morgan, P.T., M.J. Barton, and J.L. Bowtell (2019). Montmorency cherry supplementation improves 15-km cycling time-trial performance. Eur. J. Appl. Physiol. E-pub ahead of print.
Murphy, C.A., M.D. Cook, and M.E.T. Willems (2017). Effect of New Zealand blackcurrant extract on repeated cycling time trial performance. Sports 5(2):E25.
Oh, J.K., Y.O. Shin, J.H. Yoon, S.H. Kim, H.C. Shin, and H.J. Hwang (2010). Effect of supplementation with Ecklonia cava polyphenol on endurance performance of college students. Int. J. Sport Nutr. Exerc. Met. 20:72-79.
Oracz, J., D. Zyzelewicz, and E. Nebesny (2015). The content of polyphenolic compounds in cocoa beans (Theobroma cacao L.), depending on variety, growing region, and processing operations: A review. Crit. Rev. Food Sci. Nutr. 55:1176-1192.
Peake, J.M, O. Neubauer, P.A. Della Gatta, and K. Nosaka (2017). Muscle damage and inflammation during recovery from exercise. J. Appl. Physiol. 122:559-570.
Powers, S.K. and M.J. Jackson (2008). Exercise-induced oxidative stress: cellular mechanisms and impact on muscle force production. Physiol. Rev. 88:1243-1276.
Perkins, I.C., S.A. Vine, S.D. Blacker, and M.E. Willems (2015). New Zealand blackcurrant extract improves high-intensity intermittent running. Int. J. Sport Nutr. Exerc. Met. 25:487-493.
Ramirez-Sanchez, I., P.R. Taub, T.P. Ciaraldi, L. Nogueira, T. Coe, G. Perkins, M. Hogan, A.S. Maisel, R.R. Henry, G. Ceballos, and F. Villarreal (2013). (-)-Epicatechin rich cocoa mediated modulation of oxidative stress regulators in skeletal muscle of heart failure and type 2 diabetes patients. Int. J. Cardiol. 168:3982-3990.
Richards, J.C., M.C. Lonac, T.K. Johnson, M.M. Schweder, and C. Bell (2010). Epigallocatechin-3- gallate increases maximal oxygen uptake in adult humans. Med. Sci. Sports Exerc. 42:739- 744.
Roelofs, E.J., A.E. Smith-Ryan, E.T. Trexler, K.R. Hirsch, and M.G. Mock (2017). Effects of pomegranate extract on blood flow and vessel diameter after high-intensity exercise in young, healthy adults. Eur. J. Sport Sci. 17:317-325.
Trexler, E.T., A.E. Smith-Ryan, M.N. Melvin, E.J. Roelofs, and H.L. Wingfield (2014). Effects of pomegranate extract on blood flow and running time to exhaustion. Appl. Physiol. Nutr. Metab. 39:1038-1042.
Trinity, J.D., M.D. Pahnke, J.R. Trombold, and E.F. Coyle (2014). Impact of polyphenol antioxidants on cycling performance and cardiovascular function. Nutrients 6:1273-1292.
Trombold, J.R., J.N. Barnes, L. Critchley, and E.F. Coyle (2010). Ellagitannin consumption improves strength recovery 2-3 d after eccentric exercise. Med. Sci. Sports Exerc. 42:493-498.
Trombold, J.R., A.S. Reinfeld, J.R. Casler, and E.F. Coyle (2011). The effect of pomegranate juice supplementation on strength and soreness after eccentric exercise. J. Strength Cond. Res. 25:1782-1788.
Willems, M.E.T., L. Cousins, D. Williams, and S.D. Blacker (2016). Beneficial effects of New Zealand blackcurrant extract on maximal sprint speed during the Loughborough intermittent shuttle test. Sports 4(3):E42.