Key Points
-
Endurance athletes are at risk of dehydration since training/competition raises sweat rates for prolonged periods of time and the ad libitum drinking rate is typically less than the sweat rate.
-
While much research has examined the effect of dehydration on endurance athletes over the past century, there is still much debate in the scientific community about the true effects on performance.
-
Studies that have examined the effect of dehydration on endurance performance (and other exercise modes) may be, at least partially, confounded by 1) not being blinded, introducing the potential for placebo and nocebo effects on performance, and 2) using uncomfortable dehydration methods that may influence performance outside of any effect of dehydration.
-
Studies have shown that it is possible to blind volunteers to their hydration status by manipulating fluid intake through intravenous saline (venous catheter) and intragastric (nasogastric tube) water intake, with the latter appearing to induce physiological and perceptual responses more consistent with normal states of dehydration and euhydration.
-
These blinded studies demonstrated that dehydration >2% body mass impaired endurance cycling performance in the heat, at least when fluid was delivered into the gastrointestinal tract. Clearly more work is needed to understand other modes of exercise and settings, but these studies support the general consensus of unblinded dehydration studies.
-
One study reported that some habituation to dehydration is possible, suggesting that if uncomfortable and unfamiliar dehydration methods are used in studies, performance may be impaired, at least partially, by the method and not necessarily the body fluid deficit.
INTRODUCTION
Much research has focused on the influence of hypohydration or dehydration on physiological and performance responses during prolonged endurance exercise, with the topic representing one of the oldest research questions in sport and exercise physiology and nutrition, dating back some 100 years or so. Despite the vast body of research, there remains much controversy and disagreement between researchers over the real effects of hydration status on performance. Much of this controversy and debate is concentrated on how the results of research studies are incorporated into practical drinking guidelines for athletes. The various views can be broadly categorized into two schools of thought: those that suggest drinking during exercise should be planned and those that suggest drinking should be ad libitum or guided by thirst (reviewed in Kenefick, 2018). Clearly, how research findings are incorporated into recommendations for athletes and/or applied practitioners is vitally important, but for recommendations to be well-informed, the research studies on which they are based need to be robust.
The goal of this Sports Science Exchange article is to briefly summarize the extensive body of literature exploring the influence of hydration on endurance, discuss why limitations in the methods used in these studies might limit the robustness of their findings, and finally describe the results of a small number of recent studies that have attempted to overcome these limitations. We will not, in this article, address the specifics of how athletes should drink during exercise, but will focus on the quality of the evidence that explores if dehydration has effects on performance. Clearly, before understanding how athletes should drink, there is a need to establish, in a robust manner, whether dehydration impairs performance at levels experienced by athletes. It is also important to ensure a clear understanding of some of the terminology used. Euhydration refers to normal body water but could be considered as being in a state of water balance, while hypohydration and hyperhydration refer to new physiological states of low and high body water, respectively. Dehydration actually refers to the process of losing body water, but even in the scientific literature is often used to refer to a state of low body water and for clarity will be used in this context here.
WATER BALANCE AND ENDURANCE EXERCISE
Underpinning the importance of water intake for endurance performance is the vital role that body water plays for the general health and well-being of athletes (and all human beings for that matter). Water is the most abundant molecule in the human body, accounting for ~60-70% of most athletes’ body mass and is the nutrient that is lost and consumed in the largest amount each day (Brouns et al., 1989). In most sedentary individuals, water balance is achieved on a day-to-day basis through physiological regulation of urine output by the kidneys to match water intake, with net balance possible on a wide variety of water intakes (Kavouras, 2019). During exercise, metabolic rate (energy expenditure) substantially increases, with ~80% of energy being produced as heat. As a consequence, sweat production increases to facilitate evaporative cooling (evaporation of sweat from the skin), to attenuate the rise in body temperature. Typical sweat losses during endurance training or competition are in the range of 1-2 L/h but can reach 3-4 L/h in some well-trained populations (Beis et al., 2012; Baker et al., 2016).
Sweat rate during a given exercise task will depend on numerous factors, but within an individual athlete will mainly depend on the intensity of the exercise, the environmental conditions (temperature, humidity, radiant heat, etc.), clothing worn, and degree of heat acclimation. The prolonged nature of many endurance training and competition scenarios means that high sweat rates can proceed for hours at a time, presenting a greater strain on body water stores. While it is theoretically possibly to ingest sufficient fluid to match sweat lost and prevent dehydration, the common observation is that many athletes (particularly endurance athletes) drink less fluid during exercise than they lose in sweat (termed involuntary dehydration). This means that over the course of an exercise bout, substantial dehydration might accrue (Cheuvront & Haymes, 2001; Sharwood et al., 2004; Zouhal et al., 2011). Even when athletes commence exercise euhydrated, as would be expected for competitive events, the net loss of water during prolonged exercise means they may be dehydrated by the end of exercise, when performance capabilities might be most important (Hanley, 2014). This involuntary dehydration seems to occur even in situations where drinks are freely available during exercise. In many endurance exercise settings (competition and training) it may be logistically (e.g., carrying sufficient fluid, inadequate availability of drink stations, etc.) or physiologically (e.g., fluid delivery from the gastrointestinal (GI) tract and GI comfort) challenging to obtain sufficient fluid intake to replace losses.
Endurance exercise therefore presents a situation in which dehydration is likely commonplace among athletes, making it important to consider the implications for endurance performance, as well as how the effects might be best mitigated in this population.
DEHYDRATION AND ENDURANCE EXERCISE PERFORMANCE
The overwhelming majority of studies report that dehydration equivalent to greater than 2% body mass leads to significant reductions in endurance exercise performance and capacity, as reflected in the most recent American College of Sports Medicine position stand on the subject (Sawka et al., 2007). Although it is now some years since this position stand, other more recent comprehensive review articles broadly reach the same conclusion (Cheuvront & Kenefick, 2014). There are a number of physiological and perceptual alterations that occur due to dehydration during exercise that cause a cascade of effects, including reduced plasma volume (Sawka et al., 2015), reduced stroke volume and cardiac output (Montain & Coyle, 1992), reduced muscle (Gonzalez-Alonso et al., 1998) and cerebral (Trangmar et al., 2014) blood flow, increased body temperature (Sawka et al., 1985), increased glycogen use (Logan-Sprenger et al., 2013), and increased perceived exertion (Funnell et al., 2019). These effects of dehydration provide many plausible mechanisms that likely act in combination to explain the reduction in endurance performance with dehydration (Figure 1). The reduction in plasma volume caused by dehydration appears to drive the negative performance consequences (Sawka et al., 2015), and the impairment appears to be exacerbated by heat exposure (Kenefick et al., 2010).
Reductions in endurance performance with dehydration are clearly evidenced by laboratory and field cross-over studies (Sawka & Noakes, 2007; Cheuvront & Kenefick, 2014; James et al., 2019), but data from competitive endurance events appear to question the strength of this evidence. A number of studies correlating acute body mass loss (a surrogate for dehydration) during an endurance event with finishing time, have found that greater body mass loss is associated with a faster finishing time (Sharwood et al., 2004; Zouhal et al., 2011), and some have interpreted this as dehydration producing a faster finishing time. Clearly, association is not causation, but if one were to speculate, it would be logical to suppose the opposite conclusion, that it is a faster finishing time that results in a greater body mass loss and greater dehydration. Faster racing will increase metabolic heat production (and consequently sweat rate) and means there is less time available to drink, as well as making drinking more difficult due to practical and GI limitations. In line with this, Dion et al. (2013) demonstrated that during a half marathon on a treadmill, running speed was positively correlated with sweat rate, but not drinking rate, meaning that as running speed increased, dehydration also increased.
Although these field data are unlikely to suggest that dehydration enhances performance, body mass losses >5% for some of the faster finishers in real-world endurance events may lead one to question whether dehydration per se really impairs real-world performance (Sharwood et al., 2004; Zouhal et al., 2011). However, it is perhaps inevitable that dehydration will accrue during competitive endurance events in well-trained athletes since, in many situations, it will not be possible for the drinking rate to keep pace with the sweat rate. As an example, data from the 2009 Dubai marathon (Beis et al., 2012) reported that the race winner (completing the marathon in 2:05:29) had an estimated sweat rate of 3.6 L/h and ingested 1.7 L of fluid during the race (~0.8 L/h), yielding an approximately 9.8% reduction in body mass over the marathon. Clearly, maintaining euhydration or even limiting dehydration to 2% in the face of such sweat rates is likely impossible, but this does not mean that dehydration is ergogenic. These observations have led some to question the validity of intervention studies (Sawka & Noakes, 2007), but an athlete completing a race quickly whilst significantly dehydrated does not mean they would not have completed it quicker if they had been less dehydrated. However, there are other methodological considerations that might mean we need to reexamine the effects of dehydration on human performance, and these will be considered in the following sections.
METHODOLOGICAL CHALLENGES/LIMITATIONS FOR STUDYING DEHYDRATION
Particular focus in recent years has centred on the methods used to assess the effects of dehydration on endurance performance. As with any research study, the specific methods used are of paramount importance for interpreting the results. However, until recently, a number of potential methodological limitations inherent in almost all studies examining dehydration and endurance exercise have been largely ignored. In our opinion, there are two major limitations that are present in dehydration studies that might limit their interpretation. First, the inability or lack of blinding hydration interventions from subjects and, second, the use of methods to induce dehydration that are both uncomfortable and unfamiliar to subjects (James et al., 2019).
Methodological Limitations: Study Blinding. In sport nutrition research (as in other research fields), blinding of conditions is vital to reduce the chances of a placebo or nocebo effect being introduced and to increase confidence in the study results (Betts et al., 2020). In almost all areas of sport nutrition research, study blinding would be a prerequisite for acceptance of the study in a scientific journal. Indeed, placebo effects are well documented in sport nutrition research (Clark et al., 2000), and the fact that many athletes believe dehydration impairs performance (Nichols et al., 2005) means that dehydration studies might be confounded by a lack of blinding. This is not a small consideration, as the validity of almost 100 years of research is in question. However, until recently, attempts to blind subjects to their hydration status during exercise had not been made. To date, six studies have now effectively blinded subjects to their hydration status to examine endurance performance, with these studies using either intravenous (Adams et al., 2019; Cheung et al., 2015; Wall et al., 2015) or intragastric (Adams et al., 2018; Funnell et al., 2019 James et al., 2017) delivery of fluid to manipulate hydration status without the subjects’ knowledge.
A mix of results have been observed in these studies, which is likely due to the different methods used to manipulate hydration status (see James et al., 2019, for detailed discussion). Wall et al. (2015) and Cheung et al. (2015) used exercise in the heat to dehydrate subjects and manipulated the volume of intravenous rehydration provided after or during a standardized cycling preload to produce euhydration or dehydration of 2-3% body mass prior to a cycling time trial. Both studies observed no difference in cycling time trial performance between euhydrated and dehydrated trials. In contrast, two subsequent studies from our laboratory (Funnell et al., 2019; James et al., 2017) used orogastric or nasogastric feeding tubes to deliver fluid directly to the stomach during a standardized cycling preload. Similar to the other two studies, manipulating water intake in these studies produced euhydration or dehydration of 2-3% body mass prior to a cycling time trial. However, in contrast, both studies observed significant (8-11%) impairments in endurance performance with dehydration (Funnell et al., 2019; James et al., 2017) (Figure 2). A third study by Adams et al. (2018), using the intragastric method, also reported impaired endurance cycling performance. In this study, the authors also provided 25 mL of water every 5 min in both euhydrated and dehydrated trials to blunt thirst in both trials. Therefore, this study suggests that the effects of dehydration are not explained by perceived thirst, but it is important to note that it does not answer the question of whether thirst affects performance. In our view, it is likely that thirst plays an important role, but it seems likely to be only part of the picture, as performance decrements were observed even when thirst was absent.
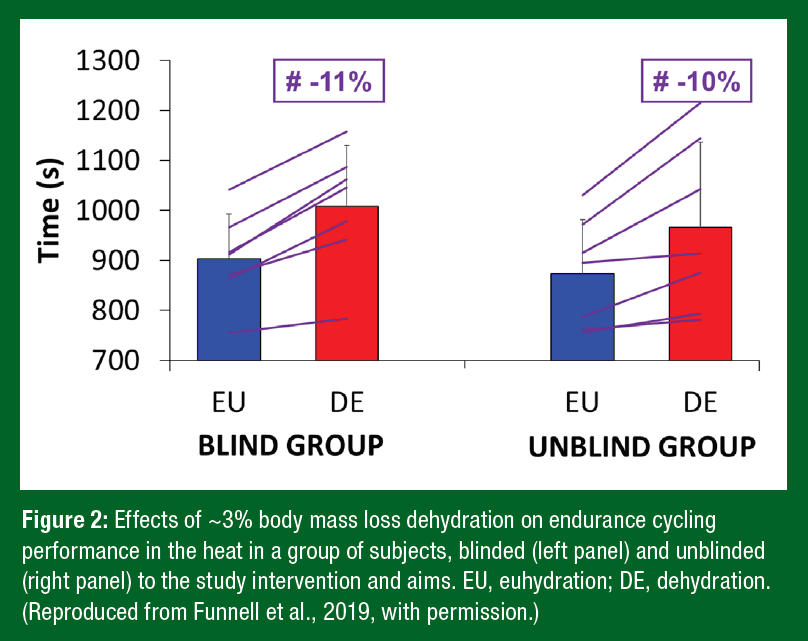
None of these studies explore the specific mechanisms by which dehydration impairs performance, but importantly, they do begin to answer the question of whether dehydration really does impair endurance performance. This begins to create a foundation from which we can build a robust evidence base for the effects of dehydration on athletic performance (although it is limited to endurance performance at this stage). The question is, why is there a clear difference in the findings? The studies that use intravenous rehydration showed no impairment in performance with dehydration, while those using intragastric rehydration did. Of course, research carried out in this way is in its infancy, and more studies are clearly needed to further examine the true effects of dehydration on endurance performance. However, it is also possible that the specific methods used also influenced the observed results. Studies using intravenous rehydration omit the interaction of the fluid with the gastrointestinal tract, which is the normal way fluid enters the body. Possibly more important is that studies using intravenous rehydration, to date, have used isotonic saline to rehydrate subjects. This means that the elevated serum osmolality, which is one of the main regulatory signals used by the body to sense hydration status, would be elevated in euhydrated and dehydrated trials alike. In contrast, those using intragastric rehydration have used water as a rehydration fluid, preventing the rise in serum osmolality, intracellular dehydration, and the subsequent cascade of induced effects. Clearly, neither method is perfect, but it seems that intragastric rehydration provides a model that replicates both physiological and perceptual responses to euhydration via oral water intake, that has not been possible (yet) with intravascular rehydration. A potentially important consideration for these blinded studies is that the studies using intragastric rehydration have delivered some fluid (albeit a small volume) orally, which has always been standardized between trials (i.e., the same volume is orally ingested in euhydrated and dehydrated trials). The activation of oropharyngeal receptors through the swallowing of fluid is potentially an important step in the fluid regulatory process (Figaro and Mack, 1997). As such, it may be that the swallowing of fluid and the consequent activation of oropharyngeal receptors is necessary to fully realize the benefit of increased hydration (James et al., 2019). In summary, these data therefore suggest that when hydration status is manipulated in a manner consistent with what athletes experience (amount and type of dehydration), endurance performance is impaired even when athletes are blinded from their hydration status.
Methodological Limitations: Uncomfortable and Unfamiliar Dehydration Methods. Real or not, the effects of dehydration observed in scientific experiments might be confounded by another factor: that being the type of methods used to induce dehydration in studies. When one considers the type of methods used to induce dehydration, they are not only overt but also atypical of the normal routine and practices of the subjects, as well as usually being uncomfortable in nature. Typical dehydration methods used in the literature involve prolonged fluid restriction (≥ 24 h), fluid restriction combined with heat exposure and/or exercise, or administration of a strong loop diuretic (e.g., furosemide). None of these are pleasant experiences and are hardly consistent with ideal preparation for athletic performance. As such, it is possible that the methods, rather than the body fluid deficit, might account for at least some of the negative performance effects of dehydration. Of particular relevance are the studies examining real-world endurance performance that reported well-trained athletes finish and even win races with significant dehydration (as determined from body mass loss over the race) (Beis et al., 2012; Sharwood et al., 2004; Zouhal et al., 2011). While dehydration might be inevitable in some settings (as discussed earlier), it is interesting to consider whether these athletes are better able to tolerate dehydration, as well as whether this is something they have always been able to do, or it is related to their experience in training/competition.
Little research is available on this topic, which is surprising given the potential significance of the results for the field of dehydration and performance. This lack of data means it is difficult to make firm conclusions. However, the one study to specifically explore the effects of repeated exposure to dehydration reported some interesting results (Fleming & James, 2014). In this study fluid intake was manipulated over 24 h (low vs. high) and during a 45-min steady state run to produce dehydration of ~2.5% body mass loss or maintain euhydration before a 5-km treadmill time trial. In the first exposure to dehydration, we observed a significant (~6%) impairment in performance, but this was reduced to a non-significant (~1%) impairment in performance after 5 dehydration exposures, although there was still a trend (P = 0.064) for a slower time in the dehydrated trial. All 10 subjects performed better dehydrated after they had been familiarized to the dehydration stimulus (Figure 3), but there was no difference in euhydrated performances when comparing before and after the dehydrated familiarizations. Although little data other than performance was collected, the changes in RPE mirrored those for performance, whereas heart rate and sweat responses were similar before and after familiarization. This suggests that the effects, at least for four sessions, are unlikely to be physiological in nature and more likely related to psychological effects. Clearly, further studies are needed to confirm these findings and explore potential mechanisms, but these data suggest some level of habituation is possible and that repeated exposure to dehydration might mean athletes are better able to tolerate it. For most endurance athletes, this repeated exposure will likely happen during training and, as such, no specific training would be required, but if not, then maybe some targeted dehydrated training sessions might be useful to expose the athlete to the levels of dehydration expected in competition with the goal of mitigating negative performance effects. The larger implications of these findings are in the interpretation of previous research examining dehydration and performance. Given that research study volunteers are rarely familiarized with the dehydration protocol used, it may mean that in some studies (perhaps temperate environments in particular), some of the reported results might be related to the dehydration protocol itself, rather than the dehydration per se.
CONCLUSIONS
Like many areas of sport nutrition research, there is still much to learn about how hydration influences endurance exercise. Hopefully, this article highlights some of the reasons why we need to carefully consider the evidence we have, particularly as it pertains to performance, as there are a number of reasons why we may need to be more cautious in our interpretation of some of the available evidence. More recent studies have attempted to overcome some of the methodological issues, and as such we are closer to having a more precise understanding of if and how dehydration impairs performance. Much of this work, at least currently, is pointing in the direction of the general scientific consensus being largely correct. To date, we can say, with some confidence at least, that dehydration > 2% body mass (produced by exercise and inadequate fluid intake) impairs endurance cycling performance even when subjects are blinded from their hydration status (Adams et al., 2018; Funnell et al., 2019; James et al., 2017). Furthermore, as Funnell et al. (2019) reported, performance responses to dehydration/euhydration were not different in groups that were blinded or unblinded to the intervention. This is, in our view, a very important finding for the interpretation of previous research looking at dehydration and performance. The implication of the methodological issues highlighted in this article is that previous work that did not blind subjects from their hydration status may have been erroneous. However, the results of Funnell et al. (2019) demonstrated that blinding was not relevant when considering endurance cycling performance in the heat at a level of dehydration equivalent to ~3% body mass, suggesting that previous research was valid. Furthermore, although only one study has been performed, this combined with the field data from competitive events suggests athletes may be able to train to build up a tolerance to dehydration, although this does not mean that dehydration will enhance performance.
Of course, there are some limitations to the conclusions we are currently able to make, and some gaps in our current understanding exist. At present, robust data with appropriate blinding is limited to dehydration > 2% body mass, cycling exercise, and only in a hot environment in males. From a mode of exercise and environmental perspective, this is the setting where we might expect to see the largest effects. In contrast to cycling (at least on a stationary bike in a laboratory), running requires the athlete to carry their own body mass and as such the mass loss associated with dehydration might mitigate some of the negative effects. Therefore, future research should seek to explore the effects of dehydration in a blinded manner in runners and in cooler environments to confirm whether there is a real performance effect in such settings. Furthermore, as with most areas of sport science/nutrition research, the effects in females should also be explored, since changes in body water, water intake, and core body temperature occur over the menstrual cycle, and future studies should seek to understand their relative effects.
PRACTICAL RECOMMENDATIONS
-
Endurance athletes should be aware that dehydration will have a negative effect on their performance, but that there is likely a high degree of variability in how much dehydration will hinder their performance.
-
Athletes should focus on two main goals related to their hydration. First, they should aim to start exercise well hydrated, which will be achieved by consuming adequate water in the days and hours leading up to an event/session. Second, they should aim to minimize dehydration that develops during exercise.
-
If possible, athletes should maintain euhydration (not losing more than 1-2% of body mass). However, it must be recognized that in some situations this will simply not be possible, and athletes should focus on ensuring any dehydration that develops is minimized.
REFERENCES
Adams, J.D., Y. Sekiguchi, H.G. Suh, A.D. Seal, C.A. Sprong, T.W. Kirkland, and S.A. Kavouras (2018). Dehydration impairs cycling performance, independently of thirst: a blinded study. Med. Sci. Sports Exerc. 50:1697-1703.
Adams, J.D., D.M. Scott, N.A. Brand, H.G. Suh, A.D. Seal, B.P. McDermott, M.S. Ganio and S.A. Kavouras (2019). Mild hypohydration impairs cycle ergometry performance in the heat: a blinded study. Scand. J. Med. Sci. Sports 29:686-695.
Baker, L.B., K.A. Barnes, M.L. Anderson, D.H. Passe, and J.R. Stofan (2016). Normative data for regional sweat sodium concentration and whole-body sweating rate in athletes. J. Sports Sci. 34:358-368.
Beis, L.Y., M. Wright-Whyte, B. Fudge, T. Noakes, and Y.P. Pitsiladis (2012). Drinking behaviors of elite male runners during marathon competition. Clin. J. Sport Med. 22:254-261.
Betts, J.A., J.T. Gonzalez, L.M. Burke, G.L. Close, I. Garthe, L.J. James, A.E. Jeukendrup, J.P. Morton, D.C. Nieman, P. Peeling, S.M. Phillips, T. Stellingwerff, L.J.C. van Loon, C. Williams, K. Woolf, R. Maughan, and G. Atkinson (2020). PRESENT 2020: Text expanding on the checklist for proper reporting of evidence in sport and exercise nutrition trials. Int. J. Sport Nutr. Exerc. Metab. 15:1-12.
Brouns, F., W.H. Saris, J. Stroecken, E. Beckers, R. Thijssen, N.J. Rehrer, and F. ten Hoor (1989) Eating drinking and cycling. A controlled Tour de France simulation study, Part 1. Int. J. Sports Med. 10:S32-S40.
Cheung, S.S., G.W. McGarr, M.M. Mallette, P.J. Wallace, C.L. Watson, I.M. Kim, and M.J. Greenway (2015). Separate and combined effects of dehydration and thirst sensation on exercise performance in the heat. Scand. J. Med. Sci. Sports. 25(Suppl 1):104-111.
Cheuvront, S.N., and E.M. Haymes (2001). Thermoregulation and marathon running: biological and environmental influences. Sports Med. 31:743-762.
Cheuvront, S.N., and R.W. Kenefick (2014). Dehydration: physiology, assessment, and performance effects. Compr. Physiol. 4:257-285.
Clark, V.R., W.G. Hopkins, J.A. Hawley, and L.M. Burke (2000). Placebo effect of carbohydrate feedings during a 40-km cycling time trial. Med. Sci. Sports Exerc. 32:1642-1647.
Dion, T., F.A. Savoie, A. Asselin, C. Gariepy, and E.D. Goulet ED (2013). Half-marathon running performance is not improved by a rate of fluid intake above that dictated by thirst sensation in trained distance runners. Eur. J. Appl. Physiol. 113:3011-3020.
Figaro MK, and G.W. Mack (1997) Regulation of fluid intake in dehydrated humans: role of oropharyngeal stimulation. Am. J. Physiol. 272:R1740–R1746.
Fleming, J., and L.J. James (2014). Repeated familiarisation with hypohydration attenuates the performance decrement caused by hypohydration during treadmill running. Appl. Physiol. Nutr. Metab. 39:124-129.
Funnell, M.P., S.A. Mears, K. Bergin-Taylor, and L.J. James (2019). Blinded and unblinded hypohydration similarly impair cycling time trial performance in the heat in trained cyclists. J. Appl. Physiol. 126:870-879.
González-Alonso, J., J.A. Calbet, and B. Nielsen (1998). Muscle blood flow is reduced with dehydration during prolonged exercise in humans. J. Physiol. 513:895-905.
Hanley, B. (2014). Senior men's pacing profiles at the IAAF World Cross Country Championships. J. Sports Sci. 32:1060-1065.
James, L.J., J. Moss, J. Henry, C. Papadopoulou, and S.A. Mears (2017). Hypohydration impairs endurance performance: a blinded study. Physiol. Rep. 5:e13315.
James, L.J., M.P. Funnell, R.M. James, and S.A. Mears (2019). Does hypohydration really impair endurance performance? Methodological considerations for interpreting hydration research. Sports Med. 49:S103-S114.
Kavouras, S.A. (2019). Hydration, dehydration, underhydration, optimal hydration: are we barking up the wrong tree? Eur. J. Nutr. 58:471-473.
Kenefick, R.W. (2018). Drinking strategies: planned drinking versus drinking to thirst. Sports Med. 48:S31-S37.
Kenefick, R.W., S.N. Cheuvront, L.J. Palombo, B.R. Ely, and M.N. Sawka (2010). Skin temperature modifies the impact of hypohydration on aerobic performance. J. Appl. Physiol. 109:79-86.
Logan-Sprenger, H.M., G.J. Heigenhauser, G.L. Jones, and L.L. Spriet (2013). Increase in skeletal-muscle glycogenolysis and perceived exertion with progressive dehydration during cycling in hydrated men. Int. J. Sport. Nutr. Exerc. Metab. 23:220-229.
Montain, S.J., and E.F. Coyle (1992). Influence of graded dehydration on hyperthermia and cardiovascular drift during exercise. J. Appl. Physiol. 73:1340-1350.
Nichols. P.E., S.S. Jonnalagadda, C.A. Rosenbloom, and M. Trinkaus (2005). Knowledge, attitudes, and behaviors regarding hydration and fluid replacement of collegiate athletes. Int. J. Sport Nutr. Exerc. Metab. 15:515-527.
Sawka, M.N., and T.D. Noakes (2007). Does dehydration impair exercise performance? Med. Sci. Sports Exerc. 39:1209-1217.
Sawka, M.N., A.J. Young, R.P. Francesconi, S.R. Muza, and K.B. Pandolf (1985). Thermoregulatory and blood responses during exercise at graded hypohydration levels. J. Appl. Physiol.59:1394-1401.
Sawka, M.N., L.M. Burke, E.R. Eichner, R.J. Maughan, S.J. Montain, and N.S. Stachenfeld (2007). American College of Sports Medicine position stand. Exercise and fluid replacement. Med. Sci. Sports Exerc. 39:377-390.
Sawka, M.N., S.N. Cheuvront, and R.W. Kenefick (2015). Hypohydration and human performance: impact of environment and physiological mechanisms. Sports Med. 45:S51-S60.
Sharwood, K.A., M. Collins, J.H. Goedecke, G. Wilson, and T.D. Noakes (2004). Weight changes, medical complications, and performance during an Ironman triathlon. Br. J. Sports Med. 38:718-724.
Trangmar, S.J., S.T. Chiesa, C.G. Stock, K.K. Kalsi, N.H. Secher, and J. González-Alonso (2014). Dehydration affects cerebral blood flow but not its metabolic rate for oxygen during maximal exercise in trained humans. J. Physiol. 592:3143-3160.
Wall, B.A., G. Watson, J.J. Peiffer, C.R. Abbiss, R. Siegel, and P.B. Laursen (2015). Current hydration guidelines are erroneous: dehydration does not impair exercise performance in the heat. Br. J. Sports Med. 49:1077–1083.
Zouhal, H., C. Groussard, G. Minter, S. Vincent, A. Cretual, A. Gratas-Delamarche, P. Delamarche, and T.D. Noakes (2011) Inverse relationship between percentage body weight change and finishing time in 643 forty-two-kilometre marathon runners. Br. J. Sports Med. 45:1101-1105.