KEY POINTS
- Increased dopaminergic and noradrenergic activity in the brain has been associated with feelings of motivation, arousal and reward and decreased fatigue, and this has been linked to enhanced performance.
- Both dopamine and norepinephrine are synthesised from the non-essential amino acid tyrosine through a shared metabolic pathway.
- Since tyrosine and the other large-neutral amino acids (LNAA) share a common transporter across the blood-brain barrier, an elevation in the plasma concentration ratio of tyrosine to LNAA increases tyrosine uptake into the brain.
- The increased delivery of tyrosine into the central nervous system may result in increased brain dopamine and norepinephrine synthesis, although this relationship may be not straightforward.
- The current balance of evidence does not support a benefit of ingesting tyrosine to enhance exercise performance, but longer-term studies may be required to reveal a clear response.
- Tyrosine supplementation does appear to consistently attenuate losses in cognitive function when individuals are exposed to challenging environments. As many sporting situations are highly dependent on effective decision-making and the successful execution of fine and gross motor skills, this would be desirable, despite no apparent benefit to physical performance.
INTRODUCTION
Fatigue is considered to be a gradual exercise-induced loss of power- or force-generating capacity of the muscles that results in the inability to maintain the required or expected power output, leading to a loss of performance in a given task (Gandevia, 2001). Early physiologists recognized that fatigue during exercise originated within the brain, while also acknowledging that events occurring within the muscles were important, meaning that the brain and periphery were intricately linked (Bainbridge, 1919; Mosso, 1904). The introduction of techniques to study changes in muscle metabolism during exercise (e.g., muscle biopsy, fuel tracers, etc.), resulted in a shift of focus toward peripheral mechanisms of fatigue. This led to a large body of literature implicating events occurring independently of the central nervous system (CNS) as key factors in the development of fatigue. This included muscle glycogen depletion and changes in the muscle metabolic environment, as well as disturbances to neuromuscular transmission and the muscle contraction process (e.g., excitation-contraction coupling). Direct evidence has since accumulated to support a clear role of the brain in fatigue, with changes in central neurotransmission, cerebral metabolism and blood flow all potentially involved (Gandevia, 2001). While a considerable amount of work is still required to elucidate the exact role and the relative contribution of these factors to the development of fatigue during exercise, access to a growing range of sophisticated techniques is gradually opening a window into the brain. It is now clear that while early scientists may have been limited in their ability to provide mechanistic insights, their observational work was remarkably insightful and remains well-founded today (Bainbridge, 1919).
One attraction of the brain neurotransmission hypothesis of central fatigue first popularized by Eric Newsholme and his team in 1987 was the possibility that the activity of these chemicals could be manipulated through changes in the diet or the provision of nutritional supplements. While this work initially focused on the neurotransmitter, serotonin (5- HT), and the possibility of altering performance through the ingestion of tryptophan (TRP) or branched-chain amino acid (BCAA) supplements, in recent years this area has shifted focus to the catecholamine neurotransmitters. The aim of this Sports Science Exchange article is to highlight the role of the catecholamines, dopamine (DA) and norepinephrine (NE), in the neurobiological mechanism of fatigue and examine whether supplementation with the amino acid catecholamine precursor tyrosine (TYR) is capable of influencing the development of central fatigue during exercise.
ROLE OF BRAIN NEUROTRANSMITTERS IN FATIGUE
The original central fatigue hypothesis proposed by Newsholme and colleagues (1987) aimed to provide a link between changes in peripheral substrate availability and the CNS, with the shift in substrate mobilization occurring during prolonged exercise directly influencing brain 5-HT synthesis. Prolonged exercise produces a marked elevation in 5-HT levels in some regions of the brain in rodents (Meeusen et al., 1996). Since increased central serotonergic activity has been associated with subjective sensations of lethargy and tiredness, Newsholme et al. (1987) suggested that this response would produce an altered perception of effort, and a loss of drive and motivation to continue exercise. While there was some early support for this hypothesis, largely inconsistent findings of many manipulation studies have made it difficult to draw any firm conclusions regarding the role of brain 5-HT in the fatigue process.
Dopamine was originally thought to only exist as the precursor to norepinephrine until it was demonstrated to act as a neurotransmitter in its own right by Nobel Prize laureate Arvid Carlsson in 1957. This quickly led Carlsson et al. (1957) to establish the role of DA in Parkinson’s disease and subsequently in motor function. The role of DA in motor function thus became a fundamental theme for research, but it is now recognized that DA is also highly involved in learning, motivation, emotion, affect and the attribution of value or salience to sensory stimuli. The brain includes several distinct DA systems and as part of the reward pathway, DA is manufactured in nerve cell bodies located within the ventral tegmental area (VTA) and is released in the nucleus accumbens and the prefrontal cortex. The motor functions of DA are linked to a separate pathway, with cell bodies in the substantia nigra that manufacture and release DA into the striatum. Every type of reward (e.g., food, exercise, gambling, etc.) has been demonstrated to influence brain DA release, and a variety of addictive drugs act by either directly or indirectly amplifying the effects of DA.
Norepinephrine, along with DA, has come to be recognized as playing a large role in attention and focus. This is perhaps not surprising given the location and distribution of NE neurons throughout the brain. They originate both in the locus coeruleus and the lateral tegmental field, and axons of the neurons in the locus coeruleus act on adrenergic receptors found in several brain regions important to emotion, motor control and alertness (e.g., amygdala, hypothalamus, striatum, thalamus). Norepinephrine neurons seem to be involved in the regulation of arousal and sleep-awake cycles as well as learning and memory, anxiety, pain, mood and brain metabolism. Norepinephrine also underlies the fight-or-flight response, increasing heart rate, triggering glucose release and increasing muscle and brain blood flow, as well as being implicated in the etiology of depression.
Prolonged exercise results in a progressive increase in tissue content of DA in a number of cerebral regions, and brain microdialysis data have demonstrated an elevation in extracellular DA and NE concentrations during exercise (Meeusen et al., 1997). While catecholaminergic neurotransmission is elevated during exercise, a series of animal studies demonstrated that a marked fall in brain tissue DA content was apparent at the point of exhaustion (Bailey et al., 1993). This has led to the suggestion that during periods of stress and bouts of strenuous exercise, there is a depletion of central catecholamines, potentially due to insufficient delivery of precursor to maintain neurotransmitter turnover (the significance of this is described below). Due to the role of DA and NE in motivation, arousal and reward this would appear likely to be an important factor in the development of fatigue during prolonged exercise.
Around the 1950s athletes began to realize that stimulants could enhance performance. This led to the widespread use of amphetamines and other stimulant drugs in many sports, with a long history of abuse in cycling events in particular. Most stimulants are closely related to DA and NE, producing a marked elevation in extracellular DA and NE concentrations through the stimulation of neurotransmitter release from storage vesicles, along with inhibition of neurotransmitter reuptake and metabolism. There is strong evidence that amphetamines can enhance the capacity to perform exercise in a dose-dependent manner (Gerald, 1978). Since the use of drugs such as amphetamine is controlled, we have been interested in the performance effects of other drugs with a similar pharmacological action. Findings from our laboratory have demonstrated that increasing catecholaminergic neurotransmission through the administration of relatively weak stimulant-like drugs can also produce improvements in exercise performance in the heat, with no apparent effect when exercise was undertaken in temperate conditions (Roelands et al., 2008; Watson et al., 2005). Further evidence for a role of DA in the development of central fatigue is provided by studies investigating intracranial stimulation of the VTA. This is a region of the brain dominated by dopaminergic projections, with selective stimulation of this area demonstrated to motivate rats to perform a variety of tasks including running ~50% longer when compared to alternative motivational techniques (Burgess et al., 1991).
DIETARY AMINO ACIDS AND NEUROTRANSMITTER PRODUCTION
The notion that constituents of the normal diet can influence aspects of brain function is not novel. Caffeine’s stimulant properties have been studied for years and there has been increasing attention on the direct and indirect effects of carbohydrate ingestion on the brain. Over the past 40 years, particular interest has been directed toward the amino acids TYR and TRP, due to their role as precursors for catecholamine and 5-HT neurotransmitter production, respectively. Administering either the amino acid itself or protein-rich meals causes plasma concentrations to rise appreciably and in proportion to the protein content of the meal (Fernstrom, 2000). While the amino acid composition of foods varies widely depending upon the source, isolated amino acid supplements are readily available from health food stores and sports nutrition suppliers. Tyrosine is found in a wide range of protein-rich dietary sources, including meat, nuts and eggs. Dairy products such as milk and cheese also contain significant quantities of TYR. Unlike some amino acids (e.g., TRP), TYR is a non-essential large-neutral amino acid (LNAA), meaning that it can also be synthesised from phenylalanine in the liver.
Under normal physiological conditions the CNS functions within a relatively stable environment, largely distinct from the compositional fluctuations occurring in the peripheral circulation. This regulation is essential to ensure normal brain function and to protect the brain from pathogens and other harmful substances. As a function of this, the capillaries supplying the brain differ structurally from those found in the periphery, forming a barrier to the entry of blood-borne substances, known as the blood-brain barrier (BBB). Large-neutral amino acids are transported into the brain by a transporter located at the BBB that is almost fully saturated at normal plasma amino acid concentrations. Uptake is competitive and shared by all the LNAAs. The ingestion of one particular LNAA causes a rapid elevation of its plasma concentration relative to the others, consequently increasing the uptake of this LNAA into brain at the expense of the remaining LNAAs. The synthesis and release in the brain of monoamines can vary directly and rapidly with changes in concentrations of their precursor amino acids, hence the interest in the study of amino acids and brain function (Fernstrom, 2000). It is worth noting, however, that the functional effects of administering amino acids are likely to be relatively small, compared with the actions produced by administering pharmaceutical drugs that enhance neurotransmitter function in the brain.
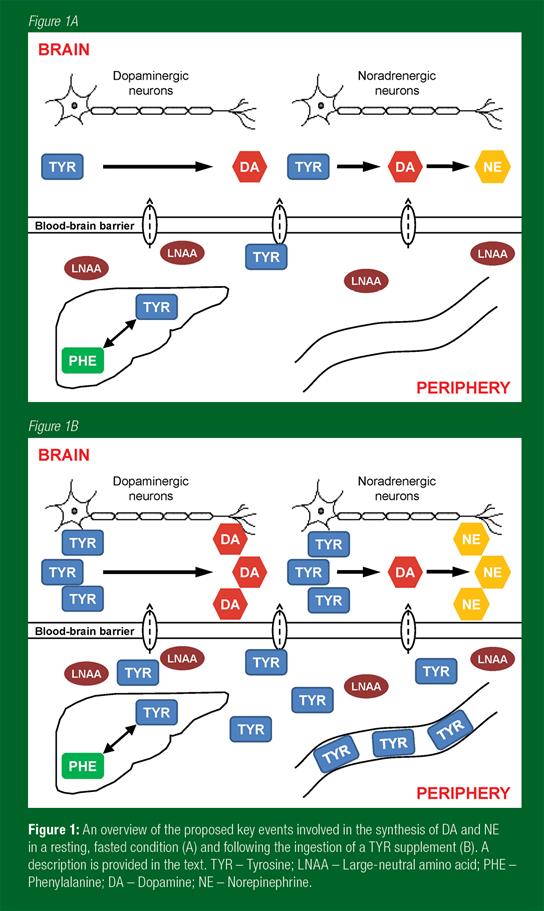
Once the TYR has reached the brain, the catecholaminergic neuro-transmitters are synthesised through a shared metabolic pathway (Figure 1A, B). Tyrosine is first hydroxylated by the enzyme tyrosine hydroxylase to levodopa (L-DOPA). A carboxyl group (-COOH) is then removed from L-DOPA by the enzyme aromatic L-amino acid decarboxylase to form DA. In noradrenergic neurons, DA is converted to NE by DA-β-hydroxylase with the addition of a hydroxyl group (-OH). Catecholamines are inactivated and metabolized by monoamine oxidase and catechol-O-methyltransferase. While there is considerable evidence of a direct relationship between the provision of TRP and the synthesis of 5-HT during both rest and exercise (Meeusen et al., 1996), the picture regarding TYR and catecholamine synthesis is less clear. It appears that this effect occurs in actively firing neurons but not in catecholamine neurons that are quiescent or firing slowly. For example, TYR administration increased noradrenergic neurotransmission in rats exposed to periods of stress (Lehnert et al., 1984; Reinstein et al., 1984), with little effect apparent while under resting conditions (Lehnert et al., 1984). Similarly, the effects on brain DA are mixed, but a microdialysis study in rodents did report a transient rise in extracellular DA in the striatum following TYR infusion (During et al., 1989).
TYROSINE SUPPLEMENTATION; DOES IT IMPROVE ATHLETIC PERFORMANCE?
Given the clear link between increased catecholaminergic activity and performance, and the evidence suggesting that TYR ingestion may elevate brain DA under conditions of stress, it should come as no surprise that several studies have examined the influence of TYR supplementation on physical performance. One of the first studies examined the effect of a relative large dose of TYR (20 g) during a prolonged bout of cycle exercise (Struder et al., 1998). This administration immediately before and during exercise did not alter physical performance, but plasma prolactin concentrations were elevated throughout exercise suggesting that some change to the brain monoaminergic system had been induced. In addition, heart rate, oxygen uptake and a variety of plasma measures (e.g., lactate, insulin, free fatty acids, glucose) did not differ between trials. Interestingly, there was a small improvement in cognitive performance following exercise apparent during the TYR trial, compared to the placebo condition. The potential of TYR to offset decrements in cognitive function is considered below.
It has been suggested that the dose of TYR administered in this initial study may have resulted in an inhibition of DA synthesis. In response to this, a lower dose of TYR (25 mg/kg body mass (BM); ~2 g for a 75 kg person) with or without the addition of carbohydrate was examined in a group of competitive cyclists that rode at 70% VO2peak for 90 min followed by a time trial lasting ~30 min (Chinevere et al., 2002). The findings demonstrated no benefit of TYR compared to the placebo condition (PL, 34.4 ± 2.9 min; TYR, 32.6 ± 3.1 min). The addition of carbohydrate to the ingested beverages resulted in a reduced time to complete the time trial (27.2 ± 0.9 min), but there was no additive effect when TYR was co-ingested with the carbohydrate (26.1 ± 1.0 min). In a separate study, the effect of TYR ingestion on aspects of muscle strength, endurance and anaerobic power were studied (Sutton et al., 2005). Male volunteers (n = 20) completed a series of tasks after ingesting TYR (150 mg/kg BM; ~11 g for a 75 kg person) or a placebo (PL). Total power output during a stepping task (PL, 216 ± 9 W; TYR 223 ± 8 W) and handgrip muscle strength and endurance were not significantly improved by TYR ingestion.
Part of the lack of effect in published studies may relate to variations in the quantity and timing of the TYR dose administered, the exercise intensity and duration employed, but also to the environmental conditions in which exercise was performed. It has been suggested that fatigue during prolonged exercise in the heat is strongly mediated by events arising within the CNS, with evidence suggesting that hyperthermia exerts a profound effect on several aspects of CNS function including changes in brain activity and voluntary muscle activation (Nielsen & Nybo, 2003). These responses have been proposed to be a protective mechanism, accelerating feelings of fatigue and consequently limiting heat production before serious damage is caused to the body’s tissues. This view is supported by observations that provision of relatively weak catecholaminergic drugs to trained cyclists markedly improved exercise performance in warm conditions (30˚C), but no benefit was apparent when the same exercise was completed in temperate conditions (18˚C) (Roelands et al., 2008; Watson et al., 2005). Administration of these drugs prior to exercise in the heat acts on central neurotransmission to maintain motivation and arousal, enabling the subjects to continue to sustain a high power output despite reaching core temperatures normally associated with the cessation of exercise. With this in mind, it is possible that TYR may be effective when exercise is performed in hot conditions.
Recently two studies, employing very similar experimental designs, attempted to answer this question (Tumilty et al., 2011; Watson et al., 2012). These both involved cycle exercise to volitional exhaustion at a workload corresponding to ~70% VO2max, with TYR (150 mg/kg BM) or a PL provided prior to exercise. While the protocol employed by these studies was very similar, the reported outcome was very different. In the study by Tumilty et al. (2011), exercise capacity was improved by 15 ± 11% following the ingestion of TYR (80.3 ± 19.7 min) compared to PL (69.2 ± 14.0 min). While there was little supporting data provided, the authors proposed that TYR ingestion either resulted in a slower rate of rise in perceived exertion and thermal stress or that volunteers reached maximal subjective sensations at a similar rate as the PL condition, but were capable of tolerating this level of stress for longer. In contrast, the results of Watson et al. (2012) demonstrated no effect of TYR supplementation on exercise capacity in a warm environment. In this case, supplementation with TYR had no effect on exercise time to exhaustion, with times of 61.4 ± 13.7 and 60.2 ± 15.4 min recorded in the PL and TYR trials, respectively. It is difficult to rationalize the marked contrast in these findings; in both cases there was a marked increase in the serum concentration ratio of TYR to the remaining LNAAs, creating an environment that would be expected to promote increased TYR transport across the BBB. It is worth noting that a follow-up study, using a pre-loaded cycle time trial protocol, reported no difference in exercise performance between trials when a TYR (35.2 ± 8.3 min) or PL (34.8 ± 6.5 min) beverage was ingested prior to exercise (Tumilty et al., 2014).
TYROSINE AND MENTAL PERFORMANCE
While evidence for an effect of TYR on physical performance is limited, TYR supplementation does appear to prevent declines in various aspects of cognitive performance and mood associated with both acute and chronic exposure to stress. There is some evidence that vigilance, choice reaction time, pattern recognition, coding and complex behaviours (such as map-compass reading), are improved by TYR administration when volunteers are exposed to the combination of cold and high altitude (Owasoyo et al., 1992). There are also several reports indicating that TYR ingestion improves stress-induced cognitive and behavioural deficits, in particular working memory and attentional focus tasks (Banderet & Lieberman, 1989; Deijen et al., 1999; Neri et al., 1995; Shurtleff et al., 1994). As many sporting situations are highly dependent upon effective decision-making and the successful execution of fine and gross motor skills, the possibility that TYR ingestion may attenuate a loss in cognitive function occurring during the later stages of exercise would be desirable, despite no apparent benefit to physical performance. This is certainly an area that warrants further investigation.
Why the Mixed Results?
As described above, there is certainly a sound rationale for TYR supplementation producing improvements to both physical and mental performance, particularly when exercise is undertaken in challenging environments, so why are mixed findings reported in the literature?
Catecholamine production in the CNS is tightly regulated through changes in the activity of tyrosine hydroxylase (TH), meaning that it is difficult to encourage the brain to produce more DA or NE. A multitude of factors govern TH activity to varying degrees, including neuronal firing rate, the impulse flow through the nigrostriatal pathway, and the amount of DA/NE synthesized (end-product inhibition) and released (feedback inhibition). While there is limited evidence that the provision of additional TYR may result in a transient increase in the rate of DA synthesis (During et al., 1989), under basal conditions cerebral levels of TYR are in excess of the concentration required for 50% of the maximal reaction velocity for TH. Consequently, the uptake of additional TYR across the BBB, resulting from an increase in the plasma concentration ratio of TYR to the other LNAAs, should not result in any significant change in the rate of DA/NE synthesis (Lehnert et al., 1984). Receptor-mediated feedback mechanisms are also likely to limit the magnitude and duration of any effect, with larger doses of TYR likely to activate this response to a greater degree (During et al., 1989). This would explain the relative lack of efficacy of catecholamine precursors in the treatment of disorders such as attention deficit hyperactivity disorder, narcolepsy and depression, while these are successfully managed by catecholamine drugs that act through increasing extracellular neurotransmitter concentrations by an alternative mechanism (e.g., reuptake inhibition).
While increasing catecholamine production is limited, limiting precursor availability does appear to produce marked effects on brain neurotransmission. As TH is not fully saturated with substrate (Carlsson & Lindqvist, 1978), the above considerations would predict that reducing brain TYR concentrations might elicit a large reduction in catecholamine biosynthesis. This is consistent with the observations where the administration of BCAA, which compete with TYR for uptake across the BBB, appeared to reduce catecholamine synthesis and release (Choi et al., 2013; Watson et al., 2004). Also in situations where catecholamine turnover is elevated for prolonged periods of time, with little opportunity for TYR ingestion (e.g., sustained military operations), reduced cerebral TYR availability might be expected to cause a depletion of brain catecholamines, consequently resulting in a loss of drive and motivation to continue. This is consistent with the hypothesis proposed by Bailey et al. (1993) and may explain the greater success of TYR supplementation in these more challenging scenarios.
CONSIDERATIONS
As with many nutritional interventions, many of these studies report a degree of variance in response to supplementation. While this is typical of human studies, some of the variance in performance may be explained by inter-individual differences in the sensitivity of neurotransmitter systems to manipulation. Studies within the neuropharmacology field have identified distinct differences in sensitivity of brain receptors between individuals, and these go some way to explaining the response to treatment in those with depression and other psychiatric disorders. While it is impossible to draw firm conclusions, it is possible that these observations may also extend to the response observed following nutritional manipulation of neurotransmission.
A major limitation when interpreting the findings of precursor loading/ manipulation studies is the degree of uncertainty regarding the fate of cerebral uptake. This makes it almost impossible to evaluate whether the manipulations have produced the desired effect on the cerebral TYR balance and whether changes in precursor uptake translate into functional alterations to extracellular neurotransmitter release. Animal models are used extensively in the neurosciences, but translating these findings to humans is complicated due to species differences in the regulation of neurotransmission and uncertainty over the correct translation of dosages. Several neurotransmitters are involved in the regulation of prolactin, adrenocorticotropic hormone, cortisol and growth hormone secretion, and as such changes in the circulating concentrations of these hormones have been employed as an index of central neurotransmission. These relationships are not straightforward, particularly during exercise, making interpretation of these hormonal changes difficult. Improvements in imaging techniques (e.g., positron emission topography) will improve our understanding of the role of changes in brain neurochemistry in the fatigue process.
It is also worth noting that it is unclear whether regular supplementation with the quantities of TYR provided in many studies (e.g., 5-10 g/day) would result in any adverse health effects due to chronic changes in sympathetic nervous system activity.
SUMMARY AND PRACTICAL APPLICATIONS
Evidence from several drug studies suggests that increased brain dopaminergic and noradrenergic activity is associated with feelings of motivation, arousal and reward and this has been linked to enhanced performance during prolonged exercise. As both dopamine and noradrenaline are synthesised from the non-essential amino acid TYR it has been suggested that TYR supplementation may increase delivery of TYR into the CNS and result in increased brain DA and NE synthesis. The implications for the sports performer remain unclear, as despite a good rationale for its use, evidence of an ergogenic benefit of TYR supplementation during prolonged exercise is limited. However, there is considerable evidence to suggest that supplementation may attenuate losses in cognitive function seen when individuals are exposed to stressful environments. Bearing this in mind, there does appear to be some potential application in situations where exercise is performed over several days in physically and mentally stressful environments. While many of the positive TYR studies have military applications, there are sporting scenarios where challenging conditions are encountered over prolonged periods of time; e.g., Tour de France, Marathon des Sables. While it can be difficult to recreate these conditions in a laboratory environment, perhaps future exercise-based TYR supplementation studies should focus on these more challenging scenarios.
REFERENCES
- Bailey, S.P., J.M. Davis, and E.N. Ahlborn (1993). Neuroendocrine and substrate responses to altered brain 5-HT activity during prolonged exercise to fatigue. J. Appl. Physiol. 74:3006-3012.
- Bainbridge, F. (1919). The physiology of muscular exercise. London: Longmans and Green.
- Banderet, L.E., and H.R. Lieberman (1989). Treatment with tyrosine, a neurotransmitter precursor, reduces environmental stress in humans. Brain Res. Bull. 22:759–762.
- Burgess, M.L., J.M. Davis, T.K. Borg and J. Buggy (1991). Intracranial self-stimulation motivates treadmill running in rats. J. Appl. Physiol. 71:1593–1597.
- Carlsson, A., M. Lindqvist, and T. Magnusson (1957). 3,4-Dihydroxyphenylalanine and 5-hydroxytryptophan as reserpine antagonists. Nature 180(4596):1200.
- Carlsson, A., and M. Lindqvist (1978) Dependence of 5-HT and catecholamine synthesis on concentrations of precursor amino acids in rat brain. Naunyn Schmied. Arch. Pharmacol. 303:157–164
- Chinevere, T.D., R.D. Sawyer, A.R, Creer, R.K. Conlee, and A.C. Parcell (2002). Effects of L-tyrosine and carbohydrate ingestion on endurance exercise performance. J. Appl. Physiol. 93:1590–1597.
- Choi, S., N. Disilvio, M.H. Fernstrom, and J.D. Fernstrom (2013). Oral branched-chain amino acid supplements that reduce brain serotonin during exercise in rats also lower brain catecholamines. Amino Acids 45:1133-1142.
- Deijen, J.B., C.J. Wientjes, H.F. Vullinghs, P.A. Cloin, and J.J. Langefeld. (1999). Tyrosine improves cognitive performance and reduces blood pressure in cadets after one week of a combat training course. Brain Res. Bull. 48:203–209.
- During, M.J., I.N. Acworth, and R.J. Wurtman (1989). Dopamine release in rat striatum: Physiological coupling to tyrosine supply. J. Neurochem. 52:1449–1454.
- Fernstrom, J.D. (2000). Can nutrient supplements modify brain function? Am. J. Clin. Nutr. 71:1669S–1673S.
- Gandevia, S.C. (2001). Spinal and supraspinal factors in human muscle fatigue. Physiol. Rev. 81:1725-1789.
- Gerald, M.C. (1978). Effects of (+)-amphetamine on the treadmill endurance performance of rats. Neuropharmacology 17:703-704.
- Lehnert, H., D.K. Reinstein, B.W. Strowbridge, and R.J. Wurtman (1984). Neurochemical and behavioral consequences of acute, uncontrollable stress: Effects of dietary tyrosine. Brain Res. 303:215–223.
- Meeusen, R., K. Thorre, F. Chaouloff, S. Sarre, K. De Meirleir, G. Ebinger, and Y. Michotte (1996). Effects of tryptophan and/or acute running on extracellular 5-HT and 5-HIAA levels in the hippocampus of food-deprived rats. Brain Res. 740:245-252.
- Meeusen, R., I. Smolders, S. Sarre S, K. de Meirleir, H. Keizer, M. Serneels, G. Ebinger, and Y. Michotte (1997). Endurance training effects on neurotransmitter release in rat striatum: an in vivo microdialysis study. Acta Physiol. Scand. 159:335-341.
- Mosso, A. (1904). Fatigue. London: Swan Sonnenschein.
- Neri, D.F., D. Wiegmann, R.R. Stanny, S.A. Shappell, A. McCardie, and D.L. McKay. (1995). The effects of tyrosine on cognitive performance during extended wakefulness. Aviat. Space Environ. Med. 66:313–319.
- Newsholme, E.A., I. Acworth, and E. Blomstrand (1987) Amino acids, brain neurotransmitters and a function link between muscle and brain that is important in sustained exercise. In: G. Benzi (ed.) Advances in Myochemistry. London: Libbey Eurotext, pp. 127– 133.
- Nielsen, B., and L. Nybo (2003) Cerebral changes during exercise in the Heat. Sports Med. 33:1-11.
- Owasoyo, J.O., D.F. Neri, and J.G. Lamberth (1992). Tyrosine and its potential use as a countermeasure to performance decrement in military sustained operations. Aviat. Space Environ. Med. 63:364-369.
- Reinstein, D.K., H. Lehnert, N.A. Scott, and R.J. Wurtman. (1984). Tyrosine prevents behavioral and neurochemical correlates of an acute stress in rats. Life Sci. 34:2225– 2231.
- Roelands, B., H. Hasegawa, P. Watson, M.F. Piacentini, L. Buyse, G. De Schutter, and R. Meeusen. (2008). The effects of acute dopamine reuptake inhibition on performance. Med. Sci. Sports Exerc. 40:879–885.
- Shurtleff, D., J.R. Thomas, J. Schrot, K. Kowalski, and R. Harford (1994). Tyrosine reverses cold-induced working memory deficit in humans. Pharmacol. Biochem. Behav. 47:935– 941.
- Struder, H.K., W. Hollmann, P. Platen, M. Donike, A. Gotzmann, and K. Weber (1998). Influence of paroxetine, branched-chain amino acids and tyrosine on neuroendocrine system responses and fatigue in humans. Horm. Metab. Res. 30:188–194.
- Sutton, E.E., M.R. Coill, and P.A. Deuster (2005). Ingestion of tyrosine: Effects on endurance, muscle strength, and anaerobic performance. Int. J. Sport Nutr. Exerc. Metab. 15:173– 185.
- Tumilty, L., G. Davison, M. Beckmann, and R. Thatcher (2011) Oral tyrosine supplementation improves exercise capacity in the heat. Eur. J. Appl. Physiol. 111:2941–2950.
- Tumilty, L., G. Davison, M. Beckmann, and R. Thatcher. (2014) Failure of oral tyrosine administration to improve exercise performance in the heat. Med. Sci. Sports Exerc. 46:1417-1425.
- Watson, P., S.M. Shirreffs, and R.J. Maughan (2004). The effect of acute branched-chain amino acid supplementation on prolonged exercise capacity in a warm environment. Eur. J. Appl. Physiol. 93:306-314.
- Watson, .P, H. Hasegawa, B. Roelands, M.F. Piacentini, R. Looverie, and R. Meeusen (2005). Acute dopamine/ noradrenaline reuptake inhibition enhances human exercise performance in warm, but not temperate conditions. J. Physiol. 565:873–883.
- Watson, P., S. Enever, A. Page, J. Stockwell, and R.J. Maughan (2012) Tyrosine supplementation does not influence the capacity to perform prolonged exercise in a warm environment. Int. J. Sport Nutr. Exerc. Metab. 22:363–373.