KEY POINTS
Altitude research and associated nutrition reviews have mainly focused on high to extreme altitudes (> 3,000 m). Recent data and associated practical nutrition recommendations are emerging from studies conducted at altitudes where athletes typically train (~1,600–2,400 m).
- Until further research on carbohydrate and fat oxidation and/or protein metabolism during exercise is conducted during the types of training undertaken by athletes at low to moderate altitudes (~1,600–2,400 m), guidelines for altered macronutrient needs remain theoretical and should align with sea-level recommendations.
- It is not clear whether low to moderate altitudes have alternative effects on energy availability (EA) requirements and/or risk for Relative Energy Deficiency in Sport, but there are several recent studies to suggest that EA will play an important role in optimizing hypoxic adaptations.
- The micronutrient iron is especially important at altitude, as hypoxia results in a distinct environment where the erythropoietic drive increases reticulocyte formation and hemoglobin mass gains, which are dependent upon adequate iron availability.
- A common theme that has emerged is that more research in physiology, metabolism and nutrition should focus on low to moderate altitudes that elite athletes use for training.
INTRODUCTION
The implementation of altitude training to optimize elite athlete performance rose to prominence prior to and during the 1968 Mexico City Olympics (held at 2,250 m above sea level). Since this time, there has been an explosion in the utilization of altitude, as well as the supporting science, in the preparation of elite athletes for competition (Mujika et al., 2019). By extension, given the altered hypoxic environment and resultant altered metabolism, there are many unique nutrition-based recommendations required to optimize altitude adaptations. However, most of the research and the associated nutrition reviews have focused on high to extreme altitudes (> 3,000 m) (Bergeron et al., 2012). Therefore, the focus of this Sport Science Exchange (SSE) article will be on the novel data and associated practical nutrition recommendations that are emerging from work at low to moderate altitudes that athletes typically implement into their periodized training (~1,600–2,400 m). For a comprehensive review, see Stellingwerff et al. (2019b).
GENERAL NUTRITION AND HYDRATION RECOMMENDATIONS AT LOW TO MODERATE ALTITUDES
Many elite athletes travel to specific global altitude training locations for 2–4 week training camps, repeated several times per year, rather than reside continually at altitude (Mujika et al., 2019). Therefore, it should be recognized that when most athletes enter any “training camp” situation, regardless of the altitude, they tend to increase training loads. So regardless of the hypoxic stress, the practitioner, along with coaches and athletes, should not neglect the sea-level importance of contemporary sports nutrition guidelines. Although it is commonly accepted that altitude results in increased carbohydrate (CHO) oxidation during exercise, and thus CHO intake requirements, it should be noted that the study demonstrating this was conducted at 4,300 m (Brooks et al., 1991), and another study in females at the same altitude actually showed decreased CHO utilization (Braun et al., 2000). Therefore, until further metabolism research (CHO vs. fat oxidation, protein metabolism) is conducted during the types of training undertaken by athletes at low to moderate altitudes (~1,600–2,400 m), guidelines to address macronutrient needs remain theoretical, and should align with sea-level recommendations (Burke et al., 2019; Stellingwerff, 2013; Stellingwerff et al., 2019a).
The lower air humidity associated with most altitude environments, along with hypoxia, are also likely to increase fluid losses at rest and during training. Furthermore, there are increased respiratory water losses coupled with hypoxic-induced diuresis that can also result in significant increases in water requirements at altitude to prevent dehydration (Butterfield et al., 1992). Therefore, increased hydration diligence and hydration status monitoring should be implemented. These include monitoring urine characteristics and daily body mass (BM) changes and being proactive with fluid intake during and after training sessions and with meals. Table 1 highlights unique altitude training camp situations that may require enhanced monitoring and/or nutritional interventions for the practitioner to consider with their athletes.
EFFECTS OF ALTITUDE ON ENERGY AVAILABILITY, BODY MASS AND ADAPTATION
Energy availability (EA) is a concept reflecting the amount of energy that is available after exercise for use by other body processes (such as endocrine, immune, skeletal and reproductive systems) and is calculated as energy intake (EI) minus exercise energy expenditure (EEE) relative to fat-free mass. Optimal EA is not only an important consideration at sea level, but also while at altitude, especially given the increased stress of hypoxia. This concept of low EA has recently been coined Relative Energy Deficiency in Sports (RED-S), and can have important and significant outcomes not only on athlete health but also performance (Mountjoy et al., 2018). However, it is not clear whether low to moderate altitudes have alternative effects on EA requirements and/or increase the risk of RED-S, but there are several recent studies to suggest that EA will play an important role in optimizing hypoxic adaptations.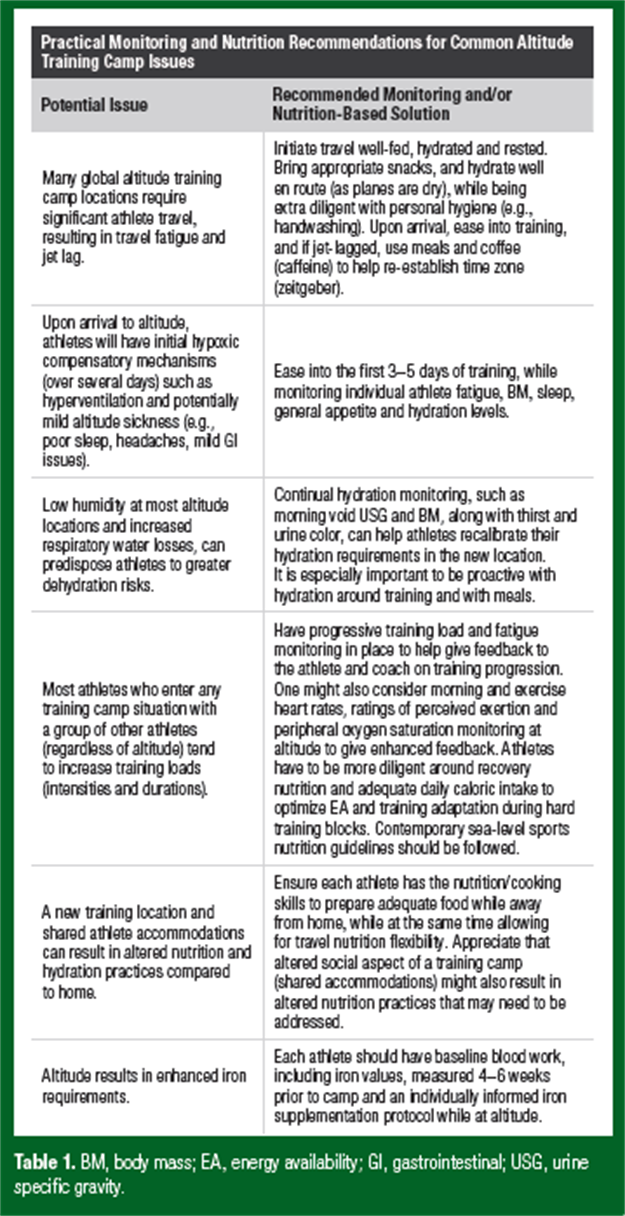
Energy Availability and Hemoglobin Mass at Altitude
Attenuation of sex hormone concentrations (estrogen, testosterone) due to low EA may impair hematological adaptations to attitude, as low EA and adverse iron metabolism have been linked (Petkus et al., 2017), and iron is a critical micronutrient to optimize hemoglobin mass (HBmass) gains at altitude (see below). Furthermore, optimization of long-term HBmass appears to be impacted by EA, as amenorrheic elite female runners had an 8% lower baseline HBmass (Figure 1) when compared to their eumenorrheic counterparts prior to an altitude camp (Heikura et al., 2018b). Interestingly, this difference in baseline HBmass was greater than the average female HBmass increase across many altitude camps (Heikura et al., 2018b) (Figure 1).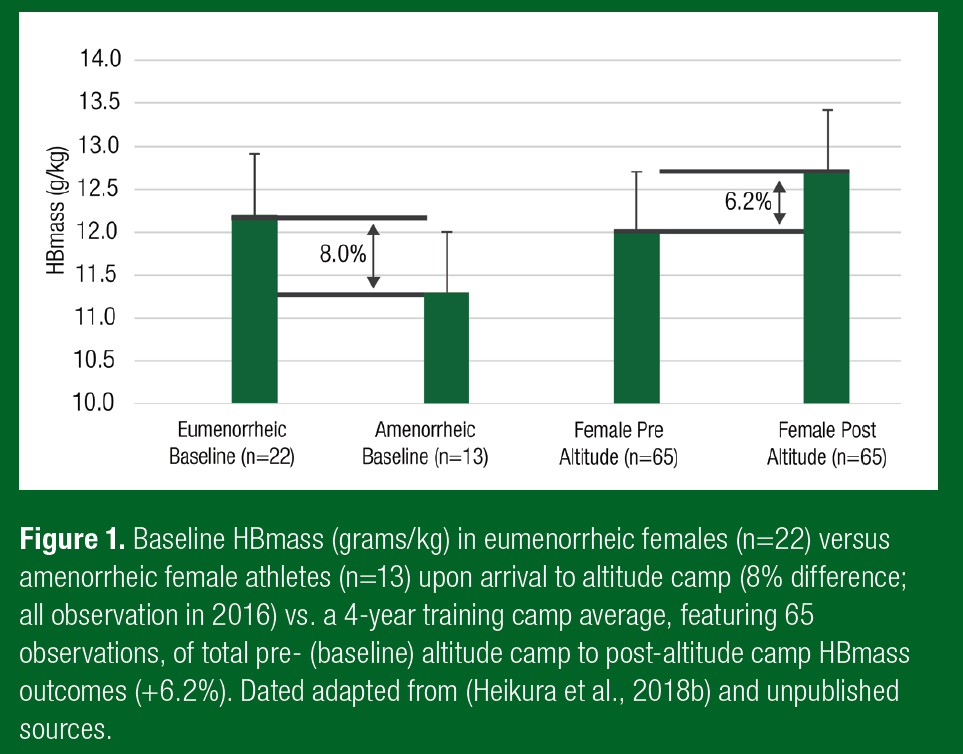
Human Metabolic Rate and Energy Intake and Expenditure Implications at Altitude
Much of the seminal research on the effects of altitude on human physiology, metabolism and associated nutritional interventions has been conducted at 4,300 m at the United States Army Pikes Peak Research Laboratory. At these high altitudes, there are consistent, and profound, effects on endocrine systems, EI, resting metabolic rate (RMR) and ultimately BM. Indeed, studies have reported 5%–15% reductions in BM, with 60%–70% of the weight loss coming via muscle atrophy at altitudes of 4,300 m (Fulco et al., 2002). The primary mechanisms for these extreme BM losses are appetite suppression resulting in lower EIs coupled with a > 3-fold increase in RMR compared to sea level (Butterfield et al., 1992). Nevertheless, aggressive EI interventions at high altitude also have shown to significantly attenuate BM losses while maintaining optimal EA (Butterfield et al., 1992).
While the effects of high-altitude exposure on EI, RMR and BM are severe, the handful of research findings at low to moderate altitudes, where athletes typically train, are much less consistent and appear to be far less deleterious. For example, a case report in four elite rowers reported loss of appetite and increased fatigue during a training block at 1,800 m (Woods et al., 2017a), while five elite runners reported an increase in appetite, with no measured change in EI, after 4 weeks of training at 2,200 m (Woods et al., 2017b). Further inconsistencies include reports of both negative (Fudge et al., 2006; Onywera et al., 2004) as well as optimal energy balance (Beis et al., 2011) in elite Kenyan and Ethiopian runners at moderate altitudes. Only two studies have investigated the effects of moderate altitude on RMR in athletes, with one study reporting a 19% increase over 4 weeks at 2,200 m in five elite runners (Woods et al., 2017b) and the second study reporting no change in RMR after 12 days at 1,800 m in four trained rowers (Woods et al., 2017a). However, given the small number of subjects, more research is required to confirm these findings that RMR is increased at low to moderate altitudes, as reported at high altitudes.
Elite Athlete Body Mass Changes at Altitude Training Camps
Given that prolonged sojourns at high altitude (> 4,000 m) can cause significant BM losses (Fulco et al., 2002), it is a commonly accepted notion that elite athletes are also at risk for significant BM losses at the typical low to moderate altitudes (~1,600–2,400 m) implemented in training camps. In terms of changes in BM during altitude training camps (~3 weeks), studies have reported no change (Beis et al., 2011; Fudge et al., 2006; Heikura et al., 2018a; Koivisto et al., 2018; Woods et al., 2017b) or minor BM decreases (Gore et al., 1998; Onywera et al., 2004; Woods et al., 2017a) when exposed to moderate altitudes. Additionally, data from four separate altitude training camps (over 3–4 weeks, with n=114 athletes) showed no relationships with BM changes (only a 0.6 ± 1.5% BM decrease), HBmass increases and injury/illness outcomes (Stellingwerff et al., 2019b). However, changes in BM alone are a poor indicator of EA status, as prolonged and/or severe reductions in EI may lead to adaptive thermogenesis, which promotes maintenance or gain of BM despite low EA (Trexler et al., 2014). Nevertheless, even though the hypoxic stress of altitudes < 2,400 m is minor in comparison with mountaineering altitudes, the extreme and prolonged training and the fact that athletes may spend as much as 20%–25% of the entire year at altitude needs to be considered (Mujika et al., 2019). Much research remains to better quantify and characterize the BM, energetic (EI, EEE and EA), metabolic and RED-S risk effects at low to moderate altitudes in athletes.
IRON — THE KEY MICRONUTRIENT CONSIDERATION FOR ALTITUDE
The micronutrient iron is especially important at altitude, as hypoxia results in a distinct environment where the erythropoietic drive increases reticulocyte formation and HBmass gains, which are dependent upon adequate iron availability. Optimal iron is especially important for endurance athletes given the importance of HBmass for aerobic power, and the fact that endurance athletes face increased iron losses through sweat, urine, the gastrointestinal tract, hemolysis (foot strike and muscle contraction), and blood loss associated with injury and menses (Sim et al., 2019). With regard to altitude, since no correlation has been observed between pre-altitude ferritin stores and the magnitude of the erythropoietic/HBmass response (Heikura et al., 2018a), it appears that iron availability via supplementation during altitude is more important for optimal adaptations than pre-altitude iron stores (Garvican-Lewis et al., 2016; Hall et al., 2019). Accordingly, it is paramount to have iron well monitored in athletes coupled with an optimal iron supplementation protocol while at altitude.
It is beyond the scope of this SSE to elucidate the extensive and complex science involving metabolism and athletes at altitude, including the various steps and considerations of iron supplementation at altitude (see the extensive reviews, Sim et al., 2019; Stellingwerff et al., 2019b). Instead, this SSE will highlight the key recommendations/steps for athletes and their staff, regarding iron screening, monitoring and supplementation with respect to altitude camps (Table 2). We also recommend the involvement of a sports medicine physician in this process, as excess iron supplementation and clinically elevated endogenous iron stores can have negative health consequences (Munoz et al., 2018).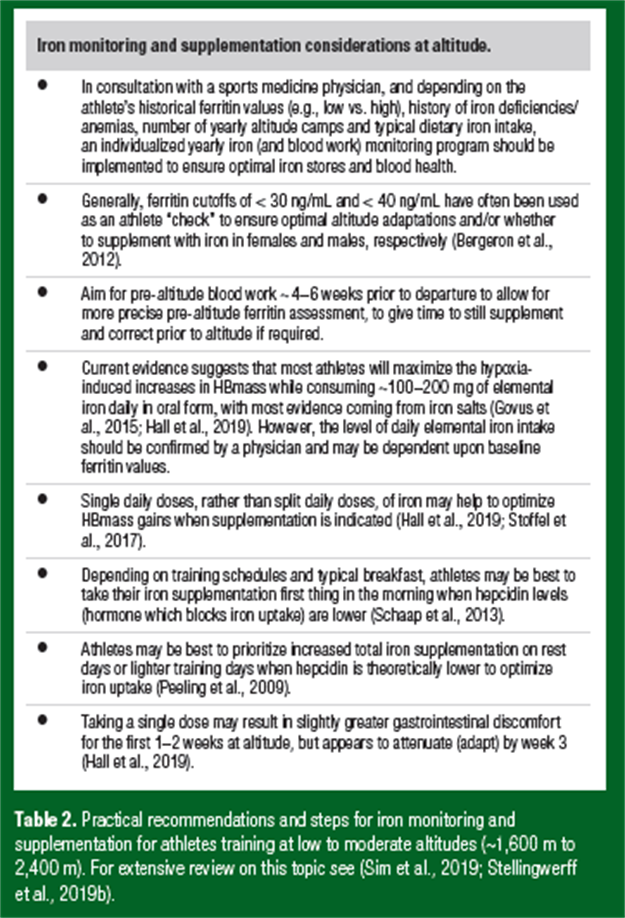
OXIDATIVE STRESS AT ALTITUDE — ARE ANTIOXIDANTS REQUIRED?
There are some emerging data on antioxidant considerations for altitude, as exercise at both extreme and moderate altitudes is associated with increased production of reactive oxygen species (ROS) with reduced antioxidant capacity, leading to oxidative stress, which may impair cell and immune function, and in some instances, potentially delaying post-exercise recovery (Sies et al., 2017). Both acute and chronic hypoxia increases oxidative stress in athletes, and this may persist for up to 2 weeks post altitude training (Pialoux et al., 2010). By extension, it might be hypothesized that since exogenous antioxidant supplements neutralize ROS, supplementation with antioxidants is needed at altitude. However, most studies have shown minimal to no effects (Subudhi et al., 2004) on ROS, and most studies have not examined the impact that exogenous antioxidants may have on training adaptations. Indeed, contemporary knowledge is that ROS actually initiates positive endurance training adaptations (Powers et al., 2010), and that single high-dose antioxidant supplementation may actually impair and attenuate training adaptation (Gomez-Cabrera et al., 2008). Interestingly, a recent study examined the impact of high and low antioxidant effects from food sources (not supplements) on the adaptive response to altitude training. This study showed that more than doubling the daily intake of antioxidant-rich foods during a 3-week altitude camp (2,320 m) did not interfere with the training responses in elite endurance athletes (measured as HBmass and maximal oxygen uptake) (Koivisto et al., 2018)). Taken together, there is not sufficient evidence to recommend high-dose single antioxidant supplementation at low to moderate altitude to attenuate altitude-induced oxidative stress. However, food-based diets high in antioxidants can be recommended, as this does not seem to attenuate training adaptation and may confer other health benefits.
PRACTICAL APPLICATIONS
Although our understanding of basic physiological and metabolic responses at low to moderate altitudes (1,600–2,400 m) compared to more extreme mountaineering altitudes is still only emerging, several practical nutrition applications can be made to further enhance altitude training adaptations (also see Tables 1, 2).
- Altitude training camps tend to present like any training camp situation with significantly increased training loads. Thus, regardless of the hypoxic stress, the practitioner should implement contemporary sea-level sports nutrition guidelines to maximize training adaptations and optimize recovery.
- Altitude locations tend to have lower air humidity and are likely to increase fluid losses at rest and during training. Therefore, increased fluid intake as well as hydration status monitoring should be implemented (Table 1).
- Although the impact of low to moderate altitude on EI, RMR and BM appear to be far less deleterious compared to extreme altitudes, some emerging indicators suggest RMR and energy requirements may be elevated. Therefore, enhanced monitoring to ensure optimal energy availability is recommended (Table 1).
- Iron is a critical micronutrient to optimize altitude adaptations. Therefore, it is paramount to have iron well monitored in athletes coupled with an optimal iron supplementation protocol while at altitude (Table 2). Current evidence suggests that most athletes will maximize the hypoxia-induced increases in HBmass while consuming ~100–200 mg of elemental iron daily in oral form, but this should be confirmed by a physician.
CONCLUSIONS
This SSE has focused on key altitude-related nutrition themes with emerging data for novel nutritional considerations at low to moderate altitudes (~1,600-2,400 m), and associated nutrition and altitude-related monitoring tools. A common theme that has emerged is that more research should focus on low to moderate altitudes that elite athletes use for training. However, recent data has shown that general contemporary nutrition guidelines, iron supplementation, and optimal energy availability are nutritional concepts that are vital to optimize altitude training adaptations.
REFERENCES
Beis, L.Y., L. Willkomm, R. Ross, Z. Bekele, B. Wolde, B. Fudge, and Y.P. Pitsiladis (2011). Food and macronutrient intake of elite Ethiopian distance runners. J. Int. Soc. Sports Nutr. 8:7.
Bergeron, M.F., R. Bahr, P. Bartsch, L. Bourdon, J.A. Calbet, K.H. Carlsen, O. Castagna, J. González-Alonso, C. Lundby, R.J. Maughan, G. Millet, M. Mountjoy, S. Racinais, P. Rasmussen, D.G. Singh, A.W. Subudhi, A.J. Young, T. Soligard, and L. Engebretsen (2012). International Olympic Committee consensus statement on thermoregulatory and altitude challenges for high-level athletes. Br. J. Sports Med. 46:770-779.
Braun, B., J.T. Mawson, S.R. Muza, S.B. Dominick, G.A. Brooks, M.A. Horning, P.B. Rock, L.G. Moore, R.S. Mazzeo, S.C. Ezeji-Okoye, and G.E. Butterfield (2000). Women at altitude: carbohydrate utilization during exercise at 4,300 m. J. Appl. Physiol. 88:246-256.
Brooks, G.A., G.E. Butterfield, R.R. Wolfe, B.M. Groves, R.S. Mazzeo, J.R. Sutton, E.E. Wolfel, and J.T. Reeves (1991). Increased dependence on blood glucose after acclimatization to 4,300 m. J. Appl. Physiol. 70:919-927.
Burke, L.M., A.E. Jeukendrup, A.M. Jones, and M. Mooses (2019). Contemporary nutrition strategies to optimize performance in distance runners and race walkers. Int. J. Sport Nutr. Exerc. Metab. 29:117-129.
Butterfield, G.E., J. Gates, S. Fleming, G.A. Brooks, J.R. Sutton, and J.T. Reeves (1992). Increased energy intake minimizes weight loss in men at high altitude. J. Appl. Physiol. 72:1741-1748.
Fudge, B.W., K.R. Westerterp, F.K. Kiplamai, V.O. Onywera, M.K. Boit, B. Kayser, and Y.P. Pitsiladis (2006). Evidence of negative energy balance using doubly labelled water in elite Kenyan endurance runners prior to competition. Br. J. Nutr. 95:59-66.
Fulco, C.S., A.L. Friedlander, S.R. Muza, P.B. Rock, S. Robinson, E. Lammi, C.J. Baker-Fulco, S.F. Lewis, and A. Cymerman (2002). Energy intake deficit and physical performance at altitude. Aviat. Space Environ. Med. 73:758-765.
Garvican-Lewis, L.A., A.D. Govus, P. Peeling, C.R. Abbiss, and C.J. Gore (2016). Iron supplementation and altitude: decision making using a regression tree. J. Sports Sci. Med. 15:204-205.
Gomez-Cabrera, M.C., E. Domenech, M. Romagnoli, A. Arduini, C. Borras, F.V. Pallardo, J. Sastre, and J. Vina (2008). Oral administration of vitamin C decreases muscle mitochondrial biogenesis and hampers training-induced adaptations in endurance performance. Am. J. Clin. Nutr. 87: 142-149.
Gore, C.J., A. Hahn, A. Rice, P. Bourdon, S. Lawrence, C. Walsh, T. Stanef, P. Barnes, R. Parisotto, D. Martin, and D. Pyne (1998). Altitude training at 2690m does not increase total haemoglobin mass or sea level VO2max in world champion track cyclists. J. Sports Sci. Med. 1:156-170.
Govus, A.D., L.A. Garvican-Lewis, C.R. Abbiss, P. Peeling, and C.J. Gore (2015). Pre-altitude serum ferritin levels and daily oral iron supplement dose mediate iron parameter and hemoglobin mass responses to altitude exposure. PLoS ONE, 10:e0135120.
Hall, R., P. Peeling, E. Nemeth, D. Bergland, W.T.P. McCluskey, and T. Stellingwerff (2019). Single versus split dose of iron optimizes hemoglobin mass gains at 2106 m altitude. Med. Sci. Sports Exerc. 51:751-759.
Heikura, I.A., L.M.Burke, D. Bergland, A.L.T. Uusitalo, A.A. Mero, and T. Stellingwerff (2018a). Impact of energy availability, health, and sex on hemoglobin-mass responses following live-high-train-high altitude training in elite female and male distance athletes. Int. J. Sports Physiol. Perform. 13:1090-1096.
Heikura, I.A., A.L.T. Uusitalo, T. Stellingwerff, D. Bergland, A.A. Mero, and L.M. Burke (2018b). Low energy availability is difficult to assess but outcomes have large impact on bone injury rates in elite distance athletes. Int. J. Sport Nutr. Exerc. Metab. 28:403-411.
Koivisto, A.E., G. Paulsen, I. Paur, I. Garthe, E. Tonnessen, T. Raastad, N.E. Bastani, J. Hallén, R. Blomhoff, and S.K. Bohn (2018). Antioxidant-rich foods and response to altitude training: A randomized controlled trial in elite endurance athletes. Scand. J. Med. Sci. Sports 28:1982- 1995.
Mountjoy, M., J.K. Sundgot-Borgen, L.M. Burke, K.E. Ackerman, C. Blauwet, N. Constantini, C. Lebrun, B. Lundy, A.K. Melin, N.L. Meyer, R.T. Sherman, A.S. Tenforde, M. Klungland Torstveit, and R. Budgett (2018). IOC consensus statement on relative energy deficiency in sport (RED-S): 2018 update. Br. J. Sports Med. 52:687-697.
Mujika, I., A.P. Sharma, and T. Stellingwerff (2019). Contemporary periodization of altitude training for elite endurance athletes: a narrative review. Sports Med. ACCEPTED.
Munoz, M., S. Gomez-Ramirez, and S. Bhandari (2018). The safety of available treatment options for iron-deficiency anemia. Expert Opin. Drug Saf. 17:149-159.
Onywera, V.O., F.K. Kiplamai, M.K. Boit, and Y.P. Pitsiladis (2004). Food and macronutrient intake of elite kenyan distance runners. Int. J. Sport Nutr. Exerc. Metab 14:709-719.
Peeling, P., B. Dawson, C. Goodman, G. Landers, E.T. Wiegerinck, D.W. Swinkels, and D. Trinder (2009). Effects of exercise on hepcidin response and iron metabolism during recovery. Int. J. Sport Nutr. Exerc. Metab. 19:583-597.
Petkus, D.L., L.E. Murray-Kolb, and M.J. De Souza (2017). The unexplored crossroads of the female athlete triad and iron deficiency: a narrative review. Sports Med. 47:1721-1737.
Pialoux, V., J.V. Brugniaux, E. Rock, A. Mazur, L. Schmitt, J.P. Richalet, P. Robach, E. Clottes, J. Coudert, N. Fellmann, and R. Mounier (2010). Antioxidant status of elite athletes remains impaired 2 weeks after a simulated altitude training camp. Eur. J. Nutr. 49:285-292.
Powers, S.K., J. Duarte, A.N. Kavazis, and E.E. Talbert (2010). Reactive oxygen species are signalling molecules for skeletal muscle adaptation. Exp. Physiol. 95:1-9.
Schaap, C.C., J.C. Hendriks, G.A. Kortman, S.M. Klaver, J.J. Kroot, C.M. Laarakkers, E.T. Wiegerinck, H. Tjalsma, M.C. Janssen, and D.W. Swinkels (2013). Diurnal rhythm rather than dietary iron mediates daily hepcidin variations. Clin. Chem. 59:527-535.
Sies, H., C. Berndt, and D.P. Jones (2017). Oxidative Stress. Ann. Rev. Biochem. 86:715-748.
Sim, M., L.A. Garvican-Lewis, G.R. Cox, A. Govus, A.K.A. McKay, T. Stellingwerff, and P. Peeling (2019). Iron considerations for the athlete: a narrative review. Eur. J. Appl. Physiol. 119:1463- 1478.
Stellingwerff, T. (2013). Contemporary nutrition approaches to optimize elite marathon performance. Int. J. Sports Physiol. Perform. 8:573-578.
Stellingwerff, T., I.M. Bovim, and J. Whitfield (2019a). Contemporary nutrition interventions to optimize performance in middle-distance runners. Int. J. Sport Nutr. Exerc. Metab. 29:106- 116.
Stellingwerff, T., P. Peeling, L.A. Garvican-Lewis, R. Hall, A.E. Koivisto, I.A. Heikura, and L.M. Burke (2019b). Nutrition and altitude: strategies to enhance adaptation, improve performance and maintain health – a narrative review. Sports Med. ACCEPTED.
Stoffel, N.U., C.I. Cercamondi, G. Brittenham, C. Zeder, A.J. Geurts-Moespot, D.W. Swinkels, D. Moretti, and M.B. Zimmermann (2017). Iron absorption from oral iron supplements given on consecutive versus alternate days and as single morning doses versus twice-daily split dosing in iron-depleted women: two open-label, randomised controlled trials. Lancet Haematol. 4:e524-e533.
Subudhi, A.W., K.A. Jacobs, T.A. Hagobian, J.A. Fattor, C.S. Fulco, S.R. Muza, P.B. Rock, A.R. Hoffman, A. Cymerman, and A.L. Friedlander (2004). Antioxidant supplementation does not attenuate oxidative stress at high altitude. Aviat. Space Environ. Med. 75:881-888.
Trexler, E.T., A.E. Smith-Ryan, and L.E. Norton (2014). Metabolic adaptation to weight loss: implications for the athlete. J. Int. Soc. Sports Nutr. 11:7.
Woods, A.L., L.A. Garvican-Lewis, A. Rice, and K.G. Thompson (2017a). 12 days of altitude exposure at 1800 m does not increase resting metabolic rate in elite rowers. Appl. Physiol. Nutr. Metab. 42:672-676.
Woods, A.L., A.P. Sharma, L.A. Garvican-Lewis, P.U. Saunders, A.J. Rice, and K.G. Thompson. (2017b). Four weeks of classical altitude training increases resting metabolic rate in highly trained middle-distance runners. Int. J. Sport Nutr. Exerc. Metab. 27:83-90.