KEY POINTS
- Females can be at increased risk of certain micronutrient deficiencies (particularly iron, calcium, vitamin D, and folate). While a ‘food first’ approach should be first considered, in some instances, supplementation may be required.
- Overall energy intake is often lower in female, compared to male athletes, and this can make reaching the recommended dietary intake (RDI) for micronutrients difficult. States of low energy availability can add further challenges.
- Iron status and regulation in female athletes may be influenced by fluctuations in ovarian hormones and menstruation blood loss. Accordingly, the RDI for iron is 2.5 times higher in females, compared to males.
- Adequate calcium intake and circulating vitamin D levels are important for sustaining bone health. In the presence of low estrogen (i.e., amenorrhea) these micronutrients are increasingly important to limit the risk of stress fractures.
- Folate has a role in the production of new red blood cells, and therefore, supplementation should be considered by female athletes planning pregnancy.
INTRODUCTION
Vitamins and minerals are essential for numerous metabolic processes in the body and are fundamental for supporting athlete health and performance. Specifically, micronutrients are involved in processes such as immune functioning, hematological adaption, energy metabolism and growth and repair, and small deficiencies can lead to large metabolic disturbances. Athletes often have higher micronutrient requirements than the general population due to the increased utilization and/or losses of micronutrients associated with exercise and training adaptation. For this reason, athletes can be at an increased risk of deficiencies, as their overall requirements typically exceed that of the recommended dietary intake (RDI). Of note, diet is the primary (and preferred) means for the body to obtain a range of vitamins and minerals. However, when requirements are high, supplements can be used to assist in maintaining vitamin and mineral concentrations within an appropriate range. Due to a variety of factors, females can be at higher risk of certain micronutrient deficiencies (McClung et al., 2014), and therefore, their nutritional requirements need additional consideration. The purpose of this Sports Science Exchange article is to review the nutritional requirements of female athletes, with specific consideration to optimizing micronutrient intake and status in this population.
WHAT MAKES FEMALES UNIQUE?
There are key biological and phenotypical attributes that make females distinct from males. Males have a greater proportional muscle and bone mass, whereas females exhibit greater fat mass and lower blood volume (and subsequently hemoglobin mass) (Blair, 2007). These characteristics can result in differences in cardiac output, aerobic capacity, thermal regulation, and muscle force production between sexes. Another key difference is the presence of different steroid hormones associated with reproduction. In males, testosterone is the primary circulating hormone, which has both reproductive and anabolic roles (Hackney, 1989); in females, the primary hormones are estrogen and progesterone (Owen, 1975). Concentrations of both estrogen and progesterone change cyclically over the course of the menstrual cycle, as well as chronically over the life span (i.e., puberty through to menopause). Other factors that cause changes to ovarian hormone concentrations include hormonal contraceptive use, pregnancy, and/or diet/exercise imbalances. Collectively, these morphological and hormonal differences between sexes make females unique, and therefore, micronutrient requirements need to consider such differences.
NUTRITIONAL CONSIDERATIONS FOR THE FEMALE ATHLETE
Currently, there is insufficient evidence to provide generalizable nutritional advice for female athletes to tailor their requirements to menstrual status or the menstrual cycle, primarily due to a lack of quality research to support any such recommendation (Holtzman & Ackerman, 2021). For instance, if we focus on just one macronutrient, carbohydrate (CHO), recent reviews found that only 11% of participants in studies examining acute fuelling strategies (Kuikman et al., 2023), and 16% of participants in studies examining chronic fuelling strategies (Kuikman et al., In Press) were female. Furthermore, of the female research that does exist, just 6.6% and 0.8% of studies examining acute and chronic fuelling strategies, respectively, used adequate methodological control of ovarian hormonal profiles (Kuikman et al., 2023; In Press). Accordingly, given the current paucity of data examining the more general nutrient requirements of female athletes, it is near impossible to provide empirically driven advice specific to menstrual status, or across the menstrual cycle.
The most practical attempt to account for females in the current sports nutrition guidelines has been to scale energy and macronutrient intake for body size (rather than absolute amounts), in recognition of the often-smaller body size of females. Accordingly, absolute energy intake requirements of female athletes are often lower than men, and this is commonly associated with lower micronutrient intake. This is counterintuitive, as the RDI of some micronutrients is either equal to or higher for women compared to men. For example, a typical Western diet has been shown to provide ~ 6 mg of dietary iron per 1,000 kcal of energy (Thomas et al., 2016). For a male to reach their recommended daily intake for iron (8 mg/day), just 1,330 kcal would be required, compared to a female who would be required to consume 3,000 kcal to reach her recommended daily intake (18 mg/day); that’s a 56% higher caloric intake! As such, consuming adequate micronutrient intake from the diet can be more challenging for females due to the dichotomy of requiring larger amounts of micronutrients from smaller energy intakes. To compound this issue, females may be more likely to adhere to special diets that can be restrictive for micronutrient intake. For example, vegetarian/vegan diets are lower in heme iron sources, which are known to have superior absorptive capacity. Furthermore, female athletes who restrict dairy products (e.g., lactose-free diets) are at an increased risk of inadequate calcium intake, which is problematic due to their greater need for calcium compared to men.
Another scenario that may lead to inadequate micronutrient availability is when athletes are in a state of low energy availability (LEA). Importantly, athletes are required to consume sufficient calories to meet their daily energy requirements and sustain normal physiological functioning. Low energy availability occurs when there is a mismatch between energy intake and energy expenditure. Prolonged LEA can lead to the development of the Female and Male Athlete Triad (Nattiv et al., 2021) and/or Relative Energy Deficiency in Sport (REDs) (Mountjoy et al., 2018), where significant negative effects on health and performance are noted. There is some evidence to suggest that LEA and its associated conditions are more prevalent in females compared to males (Jesus et al., 2021), however, this may be due to the relatively recent recognition of these conditions in male populations (Mountjoy et al., 2014; Nattiv et al., 2021), in addition to the difficulties associated with defining and measuring LEA in athletic cohorts (Burke et al., 2018). Interestingly, REDs and the Triad may be particularly unfavourable for females. For instance, a previous study has shown that bone health can be negatively impacted by short exposure to LEA (5 days at 15 kcal/kg fat-free mass in females, but not males (Papageorgiou et al., 2017)). This may imply that males are more robust towards some of the downstream effects of LEA, affording them more severe LEA exposure before physiological impairments are seen (De Souza et al., 2019).
Not only are LEA diets associated with lower micronutrient intakes (McKay et al., 2022), but there is evidence to suggest that LEA can negatively affect the regulation and absorption of some micronutrients. Indeed, LEA has been thought to be associated with increases in the iron regulatory hormone, hepcidin (McKay et al., 2020), which limits the amount of dietary iron that can be absorbed from the diet, potentially leading to poor iron status over time. Currently, the interaction between LEA and micronutrients is not well understood and is likely mediated by other factors impacted by LEA, such as ovarian hormone concentrations, macronutrient restrictions, and/or increased training loads. What is apparent is that either with or without LEA, female athletes can be at increased risk of micronutrient deficiencies. Indeed, it has been reported that iron deficiency is ~3 times more common in female (15-35%) compared to male (3-11%) athletes (Sim et al., 2019). Additionally, poor bone health is highly prevalent in female athletes (Chen et al., 2013), making adequate calcium and vitamin D intake increasingly important for this cohort. Specifically, stress fractures are estimated to be experienced by 9.7% of female athletes compared to 6.5% of their male counterparts (Chen et al., 2013). Therefore, it is important to understand an athlete’s micronutrient requirements and consider if this can be achieved via dietary optimization or whether supplementation is necessary. The following sections review the recommendations for four key micronutrients (Figure 1), and the implications for the female athlete.
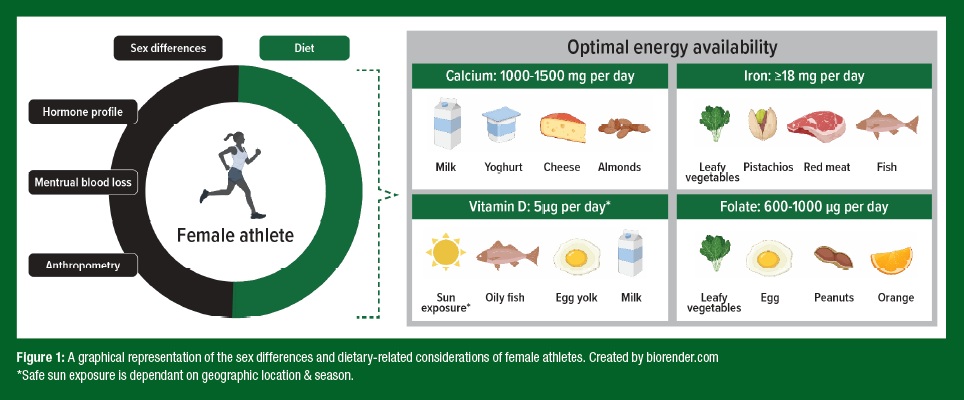
Iron
Iron is a key micronutrient that has numerous roles in the body, including oxygen transport and energy metabolism. Athletes are considered at increased risk of iron deficiency due to the increased avenues of iron loss (sweating, haemolysis, gastrointestinal bleeding) and reduced iron absorption (transient increases in hepcidin concentrations) that can result from exercise (Peeling et al., 2008). As such, it is estimated that an additional 1-2 mg iron/day is required to replenish exercise-related iron losses (Nielsen & Nachtigall, 1998) and that a higher RDI for athlete populations is likely required. When losses aren’t adequately replaced, iron deficiency, with or without anemia can eventuate. Left untreated, altered red blood cell production can impair both health and performance.
Female athletes face additional challenges when it comes to maintaining healthy iron stores. Firstly, the iron lost during menstruation can be a significant factor when considering iron balance in females, with estimates suggesting between 5-40 mg of iron is lost each cycle (Harvey et al., 2005). Furthermore, heavy menstrual bleeding (HMB) is a menstrual condition thought to be highly prevalent in female athlete cohorts (Bruinvels et al., 2016), resulting in higher iron losses and increasing an athlete’s susceptibility to iron deficiency. Of note, the use of oral contraceptive pills may assist in the management of HMB, and subsequently the management of iron deficiency. However, such approaches should only be used in consultation with a trained medical physician.
Another difference between the sexes relates to the impact of steroid hormones on the iron regulatory hormone, hepcidin. Estrogen has been shown to have inhibitory effects on hepcidin (Yang et al., 2012), which means that iron absorption may be increased during times when estrogen is elevated (i.e. phases 2 and 4 of the menstrual cycle) (Elliott- Sale et al., 2021). As such, targeted iron intake around these phases may provide an opportunity to recover the net iron loss that occurs with menstruation. However, while mechanistic support for this association is strong, studies of human trials are unclear (Alfaro-Magallanes et al., 2022; Barba-Moreno et al., 2022), and therefore, research is required to explore this idea. In males, testosterone is thought to have similar effects as estrogen by suppressing hepcidin levels (Hennigar et al., 2020). However, with testosterone concentrations remaining relatively stable day-to-day, the overall ability to absorb iron is likely to be higher in males compared to females. Collectively, these factors account for the higher rates of iron deficiency observed in female compared to male athletes (15-35% compared to 3-11%) (Sim et al., 2019). The higher iron losses observed in females are also reflected in the RDI for iron, which suggests females require 18 mg iron/day, compared to just 8 mg/iron per day in males. However, these recommendations have been created for the general population, and are likely much higher in athletic populations. Given this disparity, female athletes should be screened for iron deficiency at 6-month intervals, and more frequently (quarterly) in cases of known iron compromise, LEA, adherence to special diets, and/ or menstrual disturbances (i.e. amenorrhea). For further information, consult Sport Science Exchange article #239, which summarizes the specific demands of iron for athletes.
Calcium
When considering bone, both calcium and vitamin D have been investigated in athletes, often in the context of injury prevention (e.g., stress fracture). Specific to calcium, in the general population males and females under the age of 50 years have a similar RDI of 1000 mg/day. This increases to 1300 mg/day in postmenopausal women (50 years or older) due to lower estrogen levels; a hormone that helps to increase calcium absorption and retention in bone (Australian Government Department of Health, 2021). For athletes, an RDI of 1500 mg/d has been suggested by the International Olympic Committee (IOC) to meet the increased metabolic demands to support bone health (Mountjoy et al., 2014). Ideally, this should be spread throughout the day (in <500 mg portions) to maximize absorption (Harvey et al., 1988). It is noteworthy that this additional calcium intake is especially relevant to amenorrheic athletes to maximize the opportunity for absorption in the presence of low estrogen levels. Unsurprisingly, amenorrheic athletes are up to four times more likely to sustain a stress fracture than menstruating athletes (Heikura et al., 2018).
When considering athletic populations, prolonged intense exercise may also lead to increased loss of calcium through sweat. In combination with low dietary calcium intake, this can lower circulating calcium levels. In response, the body will seek to increase circulating calcium levels by breaking down bone (by specific cells called osteoclasts), triggered by parathyroid hormone (PTH) stimulation. Over time, this can compromise the integrity of bone and ultimately predispose the individual to osteoporosis and fracture (Rowe et al., 2022). Such events may be especially relevant to female athletes presenting with REDs. When considering adolescent females, peak bone mass is often attained by age 20 years (some increase till age 30 years) (Tan et al. In Press). Consequently, long-term exposure to LEA, especially during adolescence, may have severe long-term ramifications for bone. Specifically, early onset of osteoporosis (typically 50 years or less) predisposes an individual to fragility fractures for the rest of their life (Mäkitie & Zillikens, 2022).
Athletes can meet their calcium needs through multiple servings of dairy products (e.g. milk, yogurts, cheese) or several portions of plant-based sources daily (e.g., green leafy vegetables, broccoli, soybeans, fortified plant-based milk). Cow's milk has high calcium bioavailability with a fractional absorption rate of 32% (Weaver et al., 1999), but many plant-based foods have similar or better absorption rates (Lombardi et al., 2013). Notably, yogurt, cows’ milk, tofu and Chinese cabbage (Bok Choy) represent good sources of calcium with some of the highest absorption rates. In the event that adequate calcium intake cannot be attained from the diet alone, calcium supplements (totalling 1,000-1,500 mg/day) may be considered under the supervision of trained medical professionals. Finally, athletes should be aware of potential interactions between calcium supplements and other medications (or supplements) due to certain combinations having negative effects. For example, taking calcium and iron supplements at the same time can reduce the absorption of both minerals. Further, calcium supplements can interfere with certain medications such as antibiotics, bisphosphonates (to prevent bone loss) and thyroid hormone replacement therapy, thus compromising the medication’s effectiveness.
Vitamin D
Vitamin D is a key micronutrient that contributes to many physiological processes relevant to athletes, including skeletal muscle remodelling, muscle function, immunity, cardiac structure and function, and bone health. An estimated 33-42% of female athletes present with vitamin D insufficiency, with the type of athlete, environmental season, and geographical latitude all contributing factors (Ogan & Pritchett, 2013). The International Osteoporosis Foundation suggests circulating vitamin D levels (25-hydroxyvitamin D, 25(OH)D) between 25-49 nmol/L indicate deficiency and 75-110 nmol/L representing adequacy (International Osteoporosis Foundation, 2022)). However, there is still a large debate about what this looks like in athletes, although typically, 25(OH)D below 25 nmol/L defines deficiency (Owens et al., 2018). To date, no agreement has been reached on thresholds for insufficiency or optimal levels in athletes. Adequate sun exposure (highly dependent on geographic location/season) and dietary intake (found in oily fish, egg yolk and fortified milk) are important to optimize 25(OH)D levels. However, where inadequacies are still evident, supplementation with 2000-4000 IU vitamin D3/day may be beneficial (Owens et al., 2018). Specific to females, vitamin D also has a key role in estrogen production (Kinuta et al., 2000), and therefore, the effects of vitamin D deficiency may be wide-reaching, affecting bone health, menstrual status, and fertility (Shahrokhi et al., 2016).
One of the key roles of vitamin D is to optimize calcium absorption and bone mineralization. Accordingly, it is likely that athletes who are vitamin D deficient are at increased risk of stress fractures (Millward et al., 2020). Notably, in a study where athletes had 25(OH)D levels ≥ 75 nmol/L, approximately 30% of dietary calcium was absorbed compared to just 10-15% when 25(OH)D was < 50 nmol/L (Larson- Meyer, 2015). The close relationship between calcium and vitamin D is well established and evident by most commercial calcium supplements also containing vitamin D (cholecalciferol). With stress fractures and poor bone health particularly apparent in female athletes (Chen et al., 2013), it is important to ensure both sufficient calcium and vitamin D intake, whereby, a combined supplement may present a convenient option where co-existing deficiencies are apparent.
Folate
Folate is another vitamin that is important for female athletes. Folate is a B vitamin that has important roles in the production of new red blood cells, with a folate deficiency likely resulting in anemia and associated performance decrements. Foods such as legumes, dark leafy greens, oranges, and eggs are naturally high in folate, and in many countries’ common ingredients like wheat flour and breakfast cereals are fortified with synthetic folate (folic acid). One scenario where folate deficiency is common in females is during pregnancy, where the folate demands associated with fetal growth and development are estimated to be ~2-fold higher (McPartlin et al., 1993). This is particularly the case when an athlete continues training throughout her pregnancy, as the added hematological stress of high exercise volumes may accelerate the progression of deficiency. The RDI to support reproductive health and fetal growth is 600 μg dietary folate equivalents (DFE) of folic acid, one month prior to and during pregnancy (Australian Government Department of Health, 2021). However, these recommendations are aimed at the general population, rather than athletes, and the folate requirements for prenatal and pregnant athletes have not yet been established. However, given most prenatal vitamin supplements contain between 800-1000 μg DFE of folic acid, this should be appropriate for athletes pending a pre-existing deficiency is not evident. Nevertheless, given the lack of research in the area, individualized consultation and screening for nutrient deficiencies throughout pregnancy is highly advised. Finally, there are studies showing that oral contraceptive use is associated with reduced plasma folate and red blood cell folate concentrations (Shere et al., 2015), and therefore, athletes using oral contraception may seek to increase folate consumption, particularly if planning for pregnancy.
PRACTICAL APPLICATIONS
- Female athletes should work with their sports dietician to include nutrient-dense whole foods within their daily energy requirements.
- Supplements can have a useful role in either correcting a deficiency (i.e. iron deficiency) or ensuring adequate intake to sustain physiological processes (i.e. bone health, hematological adaption). This may be of increased importance for female athletes who have greater micronutrient losses and/or requirements.
- At this stage, no generalized nutritional guidelines can be provided to female athletes based on menstrual status and/or phase. Understanding how the menstrual cycle and hormonal contraceptive use impact nutrient regulation may assist in developing individualized strategies to minimize micronutrient deficiencies.
SUMMARY
Female athletes can be at a higher risk for micronutrient deficiencies. Ensuring adequate energy intake and a nutrient-rich diet is important in an initial ‘food first’ approach to sustaining adequate micronutrient intake. However, in certain situations, athletes may want to consider a vitamin or mineral supplement to meet their daily micronutrient needs. Iron, calcium, vitamin D and folate are identified as micronutrients which require greater attention for female athletes. When considering iron, calcium and vitamin D supplementation, females are well represented in the literature (71%), likely due to the higher rates of deficiencies evident in women (Smith et al., 2022). However, a greater understanding of menstrual status, especially in elite athletic cohorts, is still needed (Smith et al., 2022). Finally, it is important to note that for all micronutrients, supplementation will not provide an ergogenic effect and should not be used to enhance performance, rather, these supplements should only be used to correct a deficiency. Indeed, many of these micronutrients are toxic in high doses, and therefore, the decision to supplement should be taken in consultation with a trained sports dietitian.
The views expressed are those of the authors and do not necessarily reflect the position or policy of PepsiCo, Inc
REFERENCES
Alfaro-Magallanes, V.M., L. Barba-Moreno, N. Romero-Parra, B. Rael, P.J. Benito, D.W. Swinkels, C.M. Laarakkers, A.E. Diaz, A.B. Peinado, and F.S.G. Iron (2022). Menstrual cycle following interval running exercise in endurance- affects iron homeostasis and hepcidin trained women. Eur. J. Appl. Physiol. 122:2683-2694.
Australian Government Department of Health (2021). Nutrient reference values for Australia and New Zealand - Calcium. Eat for Health. Retrieved March 21st 2023, from https:// www.eatforhealth.gov.au/nutrient-reference-values
Barba-Moreno, L., V.M. Alfaro-Magallanes, X. de Jonge, A.E. Diaz, R. Cupeiro, and A.B. Peinado (2022). Hepcidin and interleukin-6 responses to endurance exercise over the menstrual cycle. Eur. J. Sport Sci. 22:218-226.
Blair, M.L. (2007). Sex-based differences in physiology: what should we teach in the medical curriculum? Adv. Physiol. Educ. 31:23-25.
Bruinvels, G., R. Burden, N. Brown, T. Richards, and C. Pedlar (2016). The prevalence and impact of heavy menstrual bleeding among athletes and mass start runners of the 2015 London Marathon. Br. J. Sports Med. 50:566-566.
Burke, L.M., B. Lundy, I.L. Fahrenholtz, and A.K. Melin (2018). Pitfalls of conducting and interpreting estimates of energy availability in free-living athletes. Int. J. Sport Nutr. Exerc. Metab. 28:350-363.
Chen, Y.T., A.S. Tenforde, and M. Fredericson (2013). Update on stress fractures in female athletes: epidemiology, treatment, and prevention. Curr. Rev. Musculoskelet. Med. 6:173-181.
De Souza, M.J., K.J. Koltun, and N.I. Williams (2019). The role of energy availability in reproductive function in the female athlete triad and extension of its effects to men: an initial working model of a similar syndrome in male athletes. Sports Med. 49(Suppl 2):125-137.
Elliott-Sale, K.J., C.L. Minahan, X.A.J. de Jonge, K.E. Ackerman, S. Sipilä, N.W. Constantini, C.M. Lebrun, and A.C. Hackney (2021). Methodological considerations for studies in sport and exercise science with women as participants: a working guide for standards of practice for research on women. Sports Med. 51:843-861.
Hackney, A.C. (1989). Endurance training and testosterone levels. Sports Med. 8:117-127.
Harvey, J.A., M.M. Zobitz, and C.Y. Pak (1988). Dose dependency of calcium absorption: a comparison of calcium carbonate and calcium citrate. J. Bone Miner. Res. 3:253–258.
Harvey, L.J., C.N. Armah, J.R. Dainty, R.J. Foxall, D.J. Lewis, N.J. Langford, and S.J. Fairweather-Tait (2005). Impact of menstrual blood loss and diet on iron deficiency among women in the UK. Br. J. Nutr. 94:557-564.
Heikura, I.A., A.L. Uusitalo, T. Stellingwerff, D. Bergland, A.A. Mero, and L.M. Burke (2018). Low energy availability is difficult to assess but outcomes have large impact on bone injury rates in elite distance athletes Int. J. Sport Nutr. Exerc. Metab. 28:403-411.
Hennigar, S.R., C.E. Berryman, M.N. Harris, J.P. Karl, H.R. Lieberman, J.P. McClung, J.C. Rood, and S.M. Pasiakos (2020). Testosterone administration during energy deficit suppresses hepcidin and increases iron availability for erythropoiesis. J. Clin. Endocrinol. Metab. 105:dgz316.
Holtzman, B., and K.E. Ackerman (2021). Recommendations and nutritional considerations for female athletes: Health and performance. Sports Med. 51:43-57.
International Osteoporosis Foundation. (2022). Vitamin D. Prevention. Retrieved March 30th 2023, from https://www.osteoporosis.foundation/health-professionals/prevention/ nutrition/vitamin-d
Jesus, F., I. Castela, A.M. Silva, P.A. Branco, and M. Sousa (2021). Risk of low energy availability among female and male elite runners competing at the 26th European cross-country championships. Nutrients 13:873.
Kinuta, K., H. Tanaka, T. Moriwake, K. Aya, S. Kato, and Y. Seino (2000). Vitamin D is an important factor in estrogen biosynthesis of both female and male gonads. Endocrinology 141:1317-1324.
Kuikman, M., E.S. Smith, A.K.A. McKay, K.E. Ackerman, R. Harris, K.J. Elliott-Sale, T. Stellingwerff, and L.M. Burke (2023). Fuelling the female athlete: auditing her representation in studies of acute carbohydrate intake for exercise. Med. Sci. Sports Exerc. 55:569-580.
Kuikman, M.A., A.K.A. McKay, E.S. Smith, K.E. Ackerman, R. Harris, K.J. Elliott-Sale, T. Stellingwerff, and L.M. Burke (In Press). Female athlete representation and dietary control methods among studies assessing chronic carbohydrate approaches to support training. Int. J. Sport Nutr. Exerc. Metab. In Press.
Larson-Meyer, D. (2015). The importance of vitamin D for athletes. Sports Sci. Exch. 28:1-8.
Lombardi, G., G. Lippi, and G. Banfi (2013). Iron requirements and iron status of athletes. The Encyclopaedia of Sports Medicine: an IOC Medical Commission publication, 19:229- 241.
Mäkitie, O., and M.C. Zillikens (2022). Early-onset osteoporosis. Calcif. Tissue Int. 110:546- 561.
McClung, J.P., E. Gaffnew-Stomberg, and J.J. Lee (2014). Female athletes: A population at risk of vitamin and mineral deficiencies affecting health and performance. J. Trace Elem. Med. Biol. 28:388-392.
McKay, A.K.A., D.B. Pyne, L.M. Burke, and P. Peeling (2020). Iron metabolism: interactions with energy and carbohydrate availability. Nutrients 12:3692.
McKay, A.K., P. Peeling, D.B. Pyne, N. Tee, J. Whitfield, A.P. Sharma, I.A. Heikura, and L.M. Burke (2022). Six days of low carbohydrate, not energy availability, alters the iron and immune response to exercise in elite athletes. Med. Sci. Sports Exerc. 54:337-387.
McPartlin, J., A. Halligan, J.M. Scott, M. Darling, and D.G. Weir (1993). Accelerated folate breakdown in pregnancy. Lancet 341:148-149.
Millward, D., A.D. Root, J. Dubois, R.P. Cohen, L. Valdivia, B. Helming, J. Kokoskie, A.L. Waterbrook, and S. Paul (2020). Association of serum vitamin D levels and stress fractures in collegiate athletes. Orthop. J. Sports Med. 8:2325967120966967.
Mountjoy, M., J. Sundgot-Borgen, L. Burke, S. Carter, N. Constantini, C. Lebrun, N. Meyer, R. Sherman, K. Steffen, and R. Budgett (2014). The IOC consensus statement: beyond the female athlete triad—Relative Energy Deficiency in Sport (RED-S). Br. J. Sports Med. 48:491-497.
Mountjoy, M., J. Sundgot-Borgen, L. Burke, K.E. Ackerman, C. Blauwet, N. Constantini, C. Lebrun, B. Lundy, A. Melin, and N. Meyer (2018). International Olympic Committee (IOC) consensus statement on Relative Energy Deficiency in Sport (RED-S): 2018 Update. Int. J. Sport Nutr. Exerc. Metab. 28:316-331.
Nattiv, A., M.J. De Souza, K.J. Koltun, M. Misra, A. Kussman, N.I. Williams, M.T. Barrack, E. Kraus, E. Joy, and M. Fredericson (2021). The male athlete triad—a consensus statement from the Female and Male Athlete Triad Coalition Part 1: Definition and scientific basis. Clin. J. Sport Med. 31:335-348.
Nielsen, P., and D. Nachtigall (1998). Iron supplementation in athletes. Current recommendations. Sports Med. 26:207-216.
Ogan, D., and K. Pritchett (2013). Vitamin D and the athlete: risks, recommendations, and benefits. Nutrients 5:1856-1868.
Owen, J.A., Jr. (1975). Physiology of the menstrual cycle. Am. J. Clin. Nutr. 28:333-338.
Owens, D.J., R. Allison, and G.L. Close (2018). Vitamin D and the athlete: Current perspectives and new challenges. Sports Med. 48:3-16.
Papageorgiou, M., K.J. Elliott-Sale, A. Parsons, J.C.Y. Tang, J.P. Greeves, W.D. Fraser, and C. Sale (2017). Effects of reduced energy availability on bone metabolism in women and men. Bone. 105:191-199.
Peeling, P., B. Dawson, C. Goodman, G. Landers, and D. Trinder. (2008). Athletic induced iron deficiency: new insights into the role of inflammation, cytokines and hormones. Eur. J. Appl. Physiol. 103:381-391.
Peeling, P., M. Sim, and A.K.A McKay. (2023). Contemporary approaches to the identification and treatment of iron deficiency in athletes. Sports Sci. Exch. #000.
Rowe, P., A. Koller, and S. Sharma (2023). Physiology, bone remodeling. In: StatPearls. StatPearls Publishing.
Shahrokhi, S.Z., F. Ghaffari, and F. Kazerouni (2016). Role of vitamin D in female reproduction. Clin. Chim. Acta 455:33-38.
Shere, M., P. Bapat, C. Nickel, B. Kapur, and G. Koren (2015). Association between use of oral contraceptives and folate status: A systematic review and meta-analysis. J. Obstet. Gynaecol. Can. 37:430-438.
Sim, M., L.A. Garvican-Lewis, G.R. Cox, A. Govus, A.K. McKay, T. Stellingwerff, and P. Peeling (2019). Iron considerations for the athlete: a narrative review. Eur. J. Appl. Physiol. 119:1463-1478.
Smith, E.S., A.K.A. McKay, M. Kuikman, K.E. Ackerman, R. Harris, K.J. Elliott-Sale, T Stellingwerff, and L.M. Burke (2022). Managing female athlete health: Auditing the representation of female versus male participants among research in supplements to manage diagnosed micronutrient issues. Nutrients 14:3372.
Tan, J., C.-A. Ng, N.H. Hart, T. Rantalainen, M. Sim, D. Scott, K. Zhu, B. Hands, and P. Chivers (2023). Reduced peak bone mass in young adults with low motor competence. J. Bone Min. Res. Online ahead of print. PMID: 36795323.
Thomas, D.T., K.A. Erdman, and L.M. Burke (2016). Position of the academy of nutrition and dietetics, dietitians of Canada, and the American College of Sports Medicine: Nutrition and athletic performance. J. Acad. Nutr. Diet. 116:501-528.
Weaver, C.M., W.R. Proulx, and R. Heaney (1999). Choices for achieving adequate dietary calcium with a vegetarian diet. Am. J. Clin. Nutr. 70:543s-548s.
Yang, Q., J. Jian, S. Katz, S.B. Abramson, and X. Huang (2012). 17β-Estradiol inhibits iron hormone hepcidin through an estrogen responsive element half-site. Endocrinology 153:3170-3178.