Key Points
- Athletes need to pay more attention to their bone health, in the short term, to reduce the risk of injury, and in the long term when they have retired from the sport.
- The general diet required by the athlete to support bone health is not markedly different from the general population.
- However, it is unclear whether dietary recommendations for the general population are sufficient to offset any deficiency or insufficiency in the athlete. For example, it is not known what the optimal intakes of various micronutrients might be to support the athlete through hard training and competition.
- If athletes do not achieve sufficient intake of certain nutrients important for bone health, or if food preferences and/or intolerances prevent or limit the intake of nutrients, athletes and practitioners could consider fortified foods or supplements (fully considering the safety aspects of doing so).
- There are some specific issues that are pertinent to the athlete including, but not necessarily limited to, energy availability, low carbohydrate availability, protein intake, vitamin D intake, and dermal calcium and sodium losses. Additional athlete specific research is, however, needed.
INTRODUCTION
What is “bone health” for the athlete? Generally, this relates to two main factors, with the primary factor in the minds of athletes and/or coaches being the avoidance of a bone injury, largely because this is the immediate danger to performance outcomes. Stress fractures are the most common bone injuries suffered by athletes; occurring relatively regularly in many sports, being caused by the rhythmic and repeated application of mechanical loading in a subthreshold manner (McBryde, 1985). Since they are overuse injuries, high-volume high-intensity training where the athlete is body-weight loaded significantly increases the risk of developing a stress fracture (Fredericson et al., 2007). These are significant injuries, and training time lost as a result can be prolonged (Ranson et al., 2010). The pathophysiology of stress fracture injuries is complex and not completely understood, but some have suggested that nutritional inadequacies could be considered a risk factor (Moran et al., 2012).
In addition to the risk of injury, there is, at least for some sports, a longer-term risk to bone health and the avoidance of osteopenia and osteoporosis becomes a consideration, particularly when bone mass is already recorded as being low in the athlete during early adulthood. This is particularly pertinent given that around 90% of peak bone mass is attained during the athlete’s early 20s. In fact, the amount of bone mass (often also referred to as bone mineral density, BMD) attained by the time an athlete is 30 years of age is about the highest they will have across the lifespan. That said, it would be erroneous to only consider bone heath in these terms. It is not only bone mass that is important but also the strength of the bone (which relates to the ability to resist the strain placed upon it), including its geometry and microarchitectural structure (relating independently to the cortical and trabecular structure of the bone). The consequences of poor bone health in later life are significant and, across Europe, the rates of osteoporotic fractures are high (Hernlund et al., 2013). Osteoporosis significantly affects not only the quality of one’s life but also quantity; for example, one-fifth of individuals requiring hospitalization for fragility fractures die within a six-month period (National Institute for Health and Clinical Excellence, 2012).
Determining long-term bone health in athletes is not necessarily straightforward, as there are no comprehensive data relating to the number of ex-athletes suffering from osteopenia or osteoporosis in older age. In addition, one could question whether it makes sense to compare athlete data to normal population data, using the T-score (that compares how much one’s bone mass deviates from the bone mass of the average healthy 30-year-old adult) or the Z-score (that compares average bone mass to people of the same age and gender). The reason for this is that many athletes would be considered smaller (e.g., marathon runners and jockeys), or larger (e.g., rugby prop forwards), than the average individual and so these general population comparisons might be misleading.
Nutrition and Bone Health
It is clear that bone can be influenced by diet and nutrition across the lifespan (Mitchell et al., 2015), as bone turnover tends to be reduced with feeding when compared to fasting (Clowes et al., 2002). When individuals are fed, both bone formation (gain) and, likely to a greater extent, bone resorption (loss) rates decrease acutely (for a good resource on this topic see: Walsh and Henriksen, 2010). This might be explained by the fact that feeding influences a number of hormones (such as calciotropic hormones, incretin hormones, growth hormone and cortisol) that in turn help to regulate bone turnover.
While it is important to know that the bone tissue can be influenced by feeding or fasting, it is just as important from a practical view to know which nutrients support the skeleton and whether athletes have bone-nutrition demands that differ from non-athletes. It stands to reason that the nutritional needs for the growing and aging skeleton of athletes will not be that different from those of the general population. Table 1 highlights some of the key nutrients to support healthy bone development (briefly summarized by Palacios, 2006). What remains to be determined is whether the hard and prolonged exercise training, often undertaken by many athletes, increases the requirement of certain nutrients over and above the amounts recommended for the general population (for examples, see the guidelines from the European Food Safety Authority, National Health and Medical Research Council, and the National Academy of Medicine). Of further consideration for the athlete, coach and practitioner is the fact that the recommended intakes are focused on making sure that the majority of the population are not deficient in a particular nutrient rather than trying to optimize function, which is one of the main concerns for the athlete (Larson-Meyer et al., 2018).
As such, some consultation with a qualified performance nutritionist or sports dietitian is advisable in order to gather a well-conducted dietary assessment related to bone health. More specifically, particular attention should be paid to the intake of dairy, fish, fruits and vegetables (particularly of the green leafy kind), since these are the most commonly cited nutrients in supporting bone health. The athlete, just like the rest of the population, will have food preferences and intolerances that might prevent or limit the intake of certain nutrients that are important for bone health. Under these circumstances, the athlete (with specialist practitioner advice and input) should consider fortified foods or supplements, particularly where a deficiency or insufficiency might also have been identified through screening.
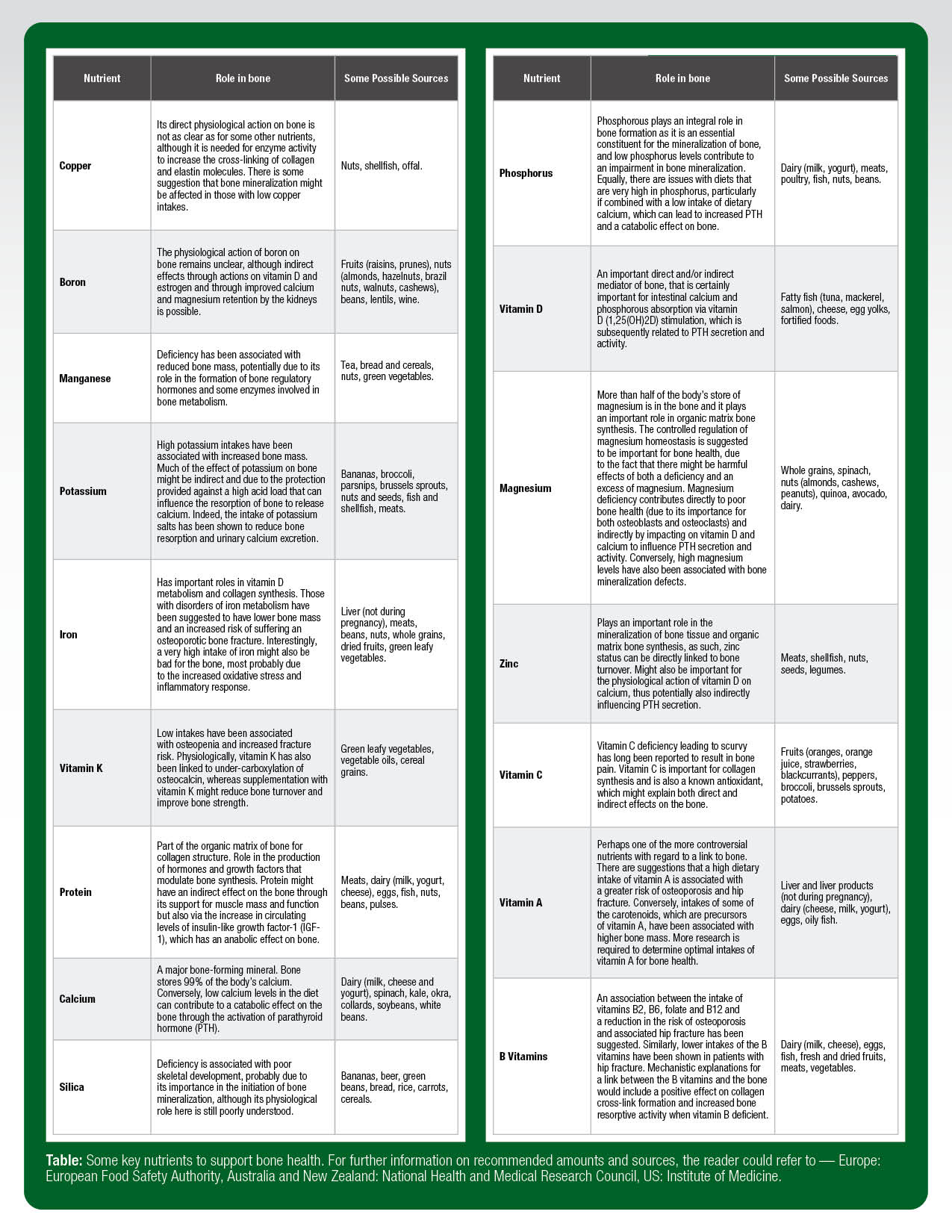
Issues Specific to the Athlete
Despite the fact that many of the dietary requirements underpinning bone health during growth and aging would be similar in the athlete to the general population, there are some dietary/nutrition challenges specific to the athlete. These include energy availability, low carbohydrate availability, protein intake, vitamin D intake, and dermal calcium and sodium losses. We currently consider these to be the main issues pertinent to the athlete, but the list is by no means exhaustive and further research might well identify further issues.
Energy Availability
Both energy balance and energy availability have been used to identify energy deficiency in physically active individuals. Energy balance is simply defined as total energy expenditure minus dietary energy intake (Figure 1), with energy availability defined as dietary energy intake minus exercise energy expenditure adjusted for muscle mass. As such, the two differ slightly in the following ways: a) the estimation of total energy expenditure, required for the determination of energy balance, may introduce more sources of errors compared to solely the measurement of exercise energy expenditure; b) energy balance assumes that bodily systems are functioning normally (but increases in exercise energy expenditure may suppress bodily functions). Therefore, in theory, individuals may be in energy balance but experience low energy availability (Papageorgiou et al., 2017).
While the link between continuous/long-term low energy availability and bone health is fairly clear and well described, particularly in female athletes, as part of the Female Athlete Triad (Nattiv et al., 2007; De Souza et al., 2014), it remains unclear whether or not the bone health of males suffers as much from the effects of low energy availability as females (Papageorgiou et al., 2017). A similar syndrome has been proposed in male athletes (Tenforde et al., 2016), which mirrors the suggestions made relating to the occurrence of impaired bone health as a result of low energy availability, by the relative energy deficiency in sport (RED-S) syndrome (Mountjoy et al., 2014; 2018).
While being in a very low energy availability state in the long term is clearly bad for bone health, it is less clear whether or not there is a level above which bone health is protected, even if this level falls below energy balance at 45 kcal·kg lean body mass (LBM)-1·d-1. There is some suggestion from the work of Ihle and Loucks (2004) that bone formation (gain) is significantly reduced at an energy availability of 30 kcal·kg LBM-1·d-1, although at this level there seems to be no increase in the amount of bone resorption (loss). It is also unclear whether there is a particular duration of low energy availability that negatively influences bone health and whether it matters if the low energy availability is continuously or intermittently applied. Of course, all of this might be very dependent upon the individual athlete. Further research is needed in this area.
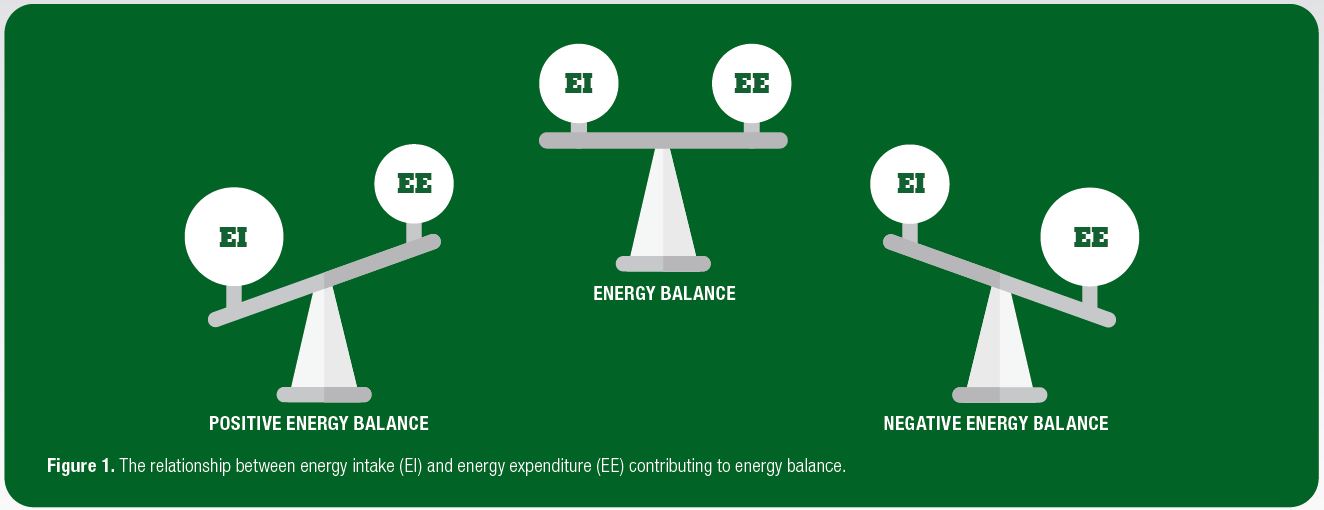
Low Carbohydrate Availability
There has been an increase in the popularity of low carbohydrate diets, or low carbohydrate-high fat diets, adopted by athletic populations in recent years. This might be, at least in part, due to the reported benefits for body composition or because it might help drive the endurance phenotype. This remains a matter of contention, given that typically carbohydrate intake provides the largest contribution to energy intake in the athlete’s diet. Furthermore, there is concern that these dietary approaches could increase the risk of a low energy availability state, which adversely affects bone health and potentially increases injury risk. There are some recent data to suggest that low carbohydrate availability might even act negatively on the bone independently of energy availability (Hammond et al., 2019), although further research is required to confirm these early findings. While no studies have examined the effects of low carbohydrate diets on bone health in athletes, Bjarnason et al. (2002) showed a reduction of ~50% in bone resorption following an oral glucose tolerance test. The provision of carbohydrate has been shown to attenuate the bone resorption response to acute exercise in athletes completing eight days of overloaded endurance training (de Sousa et al., 2014). In addition, Sale et al. (2015) have shown a post-exercise reduction in bone turnover following carbohydrate feeding during a 120 min treadmill run in recreationally active individuals. Taken together, these studies suggest that feeding carbohydrate before, during or after exercise might be a useful way to modify the bone response to hard exercise and training in the athlete, although further research is required to confirm findings. Evidence from animal models suggests that low carbohydrate diets could negatively affect bone health, particularly when followed alongside a high fat diet (Bielohuby et al., 2010). In the study by Bielohuby et al. (2010) bone mass and mechanical properties were all impaired by low-carbohydrate/high-fat diets, potentially mediated by reductions in insulin-like growth factor-1. Conversely, in humans, albeit osteoarthritis patients and not athletes, there was no effect on bone turnover when patients were fed less than 20 g/carbohydrate per day for one month and then less than 40 g/carbohydrate per day for the next two months (Carter et al., 2006).
Protein Intake
Athletes are often recommended to consume more protein than is recommended for the general population, in order to support the additional demands of athletic training (Morton et al., 2018). This presents an interesting issue in relation to bone health since it has been suggested that higher protein intakes may have an adverse effect on bone health (for a more detailed review see Dolan and Sale, 2018). In brief, the “acid-ash hypothesis” proposed that animal proteins are acidic and, as such, provide a significant challenge to the maintenance of acid-base balance in the body (Fenton et al., 2008). To protect itself, the body increases the availability of alkaline minerals, such as calcium, most of which are stored in bone (~99% in the case of calcium). This would necessitate the breakdown of bone tissue to release calcium into the circulation to counteract the effects of the increased acidity, with any excess calcium release also being lost via the urine. Over time, this process would increase the rate of bone loss and lead to a reduction in BMD (Macdonald et al., 2005). If the acid-ash hypothesis were correct, an athlete consuming a high animal-based protein diet would be at risk of negatively affecting their bone health.
The acid-ash hypothesis does not, however, provide a balanced account of the potential influence of a high protein intake on bone. Kerstetter et al. (2005) have shown that higher protein intakes result in an increase in the amount of calcium that is absorbed from foods and, as such, the increased urinary calcium levels with high animal protein intake might well come from increased calcium availability instead. Furthermore, an increased dietary acid load could just as easily occur as a result of reduced intake of alkaline foods (e.g., fruits and vegetables) as by an increase in the intake of acidic foods such as animal proteins. The flip side is that protein intake might be beneficial for the bone, given that it forms an important part of the bone structure (Zimmerman et al., 2015), and protein ingestion increases the production of a number of hormones and growth factors involved in bone formation.
Overall, it seems unlikely that higher animal protein intakes, in the amounts recommended to athletes, are harmful to bone health and, indeed, athletes might need to consume more protein than the general population to support the increased rate of bone turnover caused by athletic training. It would seem sensible to make sure that the athlete’s diet contains sufficient calcium during periods of higher protein consumption to reduce the potential for negative effects on the bone.
Vitamin D Intake
A number of studies over the last decade have identified athlete groups who have deficient or insufficient levels of circulating vitamin D (see Owens et al., 2015). Given the well-identified link between low vitamin D levels (serum 25-hydroxyvitamin D [25OHD] levels below 25 nmol·L-1) and bone, where it plays an important role in calcium and phosphorus regulation in the body, it is highly likely that athletes who are deficient in vitamin D will be at a greater risk of low bone mass (Holick, 2007) and bone injuries, such as stress fractures. A direct relationship between serum vitamin D levels and musculoskeletal outcomes is relatively clear (Scientific Advisory Committee on Nutrition, 2016) and, as such, avoiding vitamin D deficiency and insufficiency is important for the athlete to protect bone health.
Dermal Calcium Losses
Athletes who undertake a high volume of prolonged exercise might be at risk of losing calcium through sweating, which could in some circumstances reach a level that might cause a decline in serum calcium concentrations. Should this happen, a resultant increase in parathyroid hormone (PTH) secretion occurs, that would promote the breakdown of bone to release calcium into the circulation to defend the serum calcium level (Figure 2). Barry et al. (2011) suggested that supplementing with calcium before or during exercise might compensate for dermal calcium losses and defend the serum calcium level, meaning that there would be no concomitant increase in PTH release or bone resorption (Figure 2). In this study, Barry et al. (2011) showed that PTH release was less during a subsequent exercise bout when 1000 mg of calcium was ingested before exercise commenced.
Subsequent research from the Australian Institute of Sport (Haakonssen et al., 2015) has also shown that providing a calcium rich pre-exercise meal (~1350 mg) was sufficient to reduce both the PTH and bone resorption responses to a subsequent 90 min cycling bout in competitive female cyclists. Recent data have, however, suggested that dermal calcium losses are not likely to be a major cause of the increases in parathyroid hormone and bone resorption during shorter-term exercise (Kohrt et al., 2019). It is likely that the amount of dermal calcium lost in the sweat would only be significant enough to cause a disruption to calcium homeostasis and hence bone metabolism in endurance or ultra-endurance activities. It might also be of relevance for the weight making athlete using dehydration strategies to “make weight,” although this has yet to be explored.
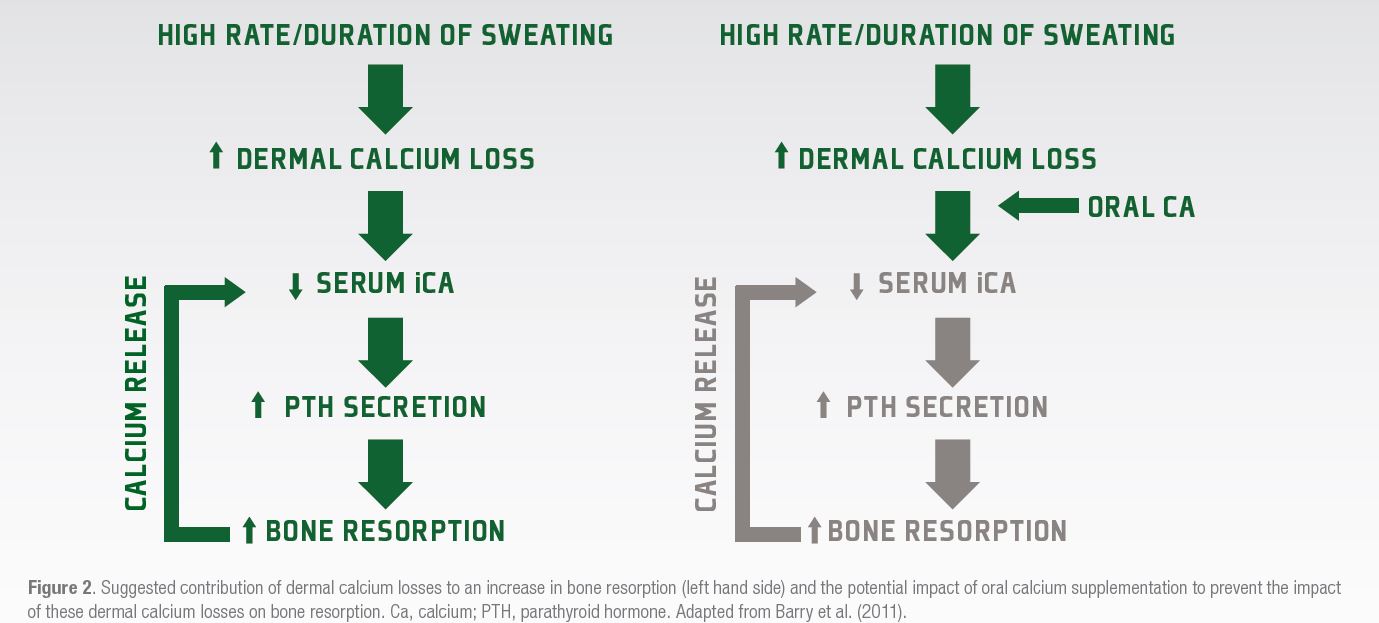
PRACTICAL APPLICATIONS
It is difficult to provide specific practical recommendations for the effects of diet on bone health in athletes. This is because the detailed evidence base, upon which to make recommendations, does not exist. Below are some “best guesses,” based upon the interpretation of the information currently available:
- Athletes should consider a well-conducted nutritional assessment of their dietary intake to identify whether or not they are consuming the required amounts of the key nutrients to underpin bone health, although this is fraught with difficulty in itself.
- Where food preferences and/or intolerances prevent or limit the intake of nutrients important for bone health, athletes and practitioners could consider fortified foods or supplements (fully considering the safety aspects of doing so).
- Energy availability of ≥ 45 kcal·kg LBM-1·d-1 is ideal to support bone health in the athlete, although this is an unrealistic target for many. Current knowledge would suggest trying to achieve an energy availability above 30 kcal·kgLBM-1·d-1 to minimize negative effects on the bone.
- Low carbohydrate diets (acutely, chronically or even periodically) might negatively influence athlete’s bone health, however, there are no studies available to provide a direct evidence base for practitioners to work from.
- Athletes often consume 2-3 times more protein than recommended daily amounts for the general population, which is now thought to have no negative effects on bone health (and possibly beneficial effects), assuming adequate dietary calcium intake (> 800 mg·d-1; also considering point 6 below).
- If an athlete is vitamin D deficient or insufficient, it is recommended that a serum vitamin D level of above 50 nmol·L-1 (20 ng·mL-1) is achieved (Institute of Medicine, 2010), although a definitive target for vitamin D in bone injury prevention remains unknown.
- Dermal calcium loss might be an important consideration for some ultra-endurance athletes, who might wish to consider increasing calcium intake before exercise.
WHAT MORE DO WE NEED TO KNOW?
- There is still a requirement to clearly define which types of athletes are, and are not, at risk of longer-term bone health issues, such as osteopenia and osteoporosis.
- Further research is needed to determine the wider implications of reduced energy availability, beyond bone, as suggested by the RED-S syndrome as currently these are not well researched.
- It remains to be clearly established whether there is a male athlete triad and whether the bone health implications of reduced energy availability are seen at the same level as in females or whether males are more resistant to the effects of low energy availability.
- It remains to be determined whether there is or is not a threshold beyond which lower energy availabilities (lower than 45 kcal·kg LBM-1·d-1) do not result in reduced bone formation.
- Further research is required into the periodization of low energy availabilities in endurance athletes, such that they can benefit from the positive effects of calorie restriction on the endurance phenotype but without putting their bone health at risk.
- More work is required in athletes to determine the effects of nutrient availability (particularly of carbohydrate) separately from energy availability on bone health.
- Although there are data available to suggest that pre-exercise calcium consumption and/or supplementation can reduce the bone resorption response to endurance exercise, the chronic effects of this strategy on bone mass and strength are not yet known.
- The amounts of calcium lost during training in endurance and ultra-endurance athletes are still not well known. Also, the amount of calcium lost during more passive sweating, particularly in hot environments, which might be performed by weight-making athletes is also not well known.
- Longer-term studies are needed to determine whether the shorter term or acute responses of bone metabolism to feeding are positive for bone health. These studies should also determine whether feeding should be periodized around hard training blocks rather than constant, so as not to reduce the potential adaptation of the bone to exercise training.
References
Barry, D.W., K.C. Hansen, R.E. van Pelt, M. Witten, P. Wolfe, and W.M. Kohrt (2011). Acute calcium ingestion attenuates exercise-induced disruption of calcium homeostasis. Med. Sci. Sports Exerc. 43:617-623.
Bielohuby, M., M. Matsuura, N. Herbach, E. Kienzle, M. Slawik, A. Hoeflich, and M. Bidlingmaier (2010). Short-term exposure to low-carbohydrate, high-fat diets induces low bone mineral density and reduces bone formation in rats. J. Bone Miner. Res. 25:275-284.
Bjarnason, N.H., E.E. Henriksen, P. Alexandersen, S. Christgau, D.B. Henriksen, and C. Christiansen (2002). Mechanism of circadian variation in bone resorption. Bone 30:307–313.
Carter, J.D., F.B. Vasey, and J. Valeriano (2006). The effect of a low-carbohydrate diet on bone turnover. Osteoporos. Int. 17:1398-1403.
Clowes, J.A., R.A. Hannon, T.S. Yap, N.R. Hoyle, A. Blumsohn, and R. Eastell (2002). Effect of feeding on bone turnover markers and its impact on biological variability of measurements. Bone 30:886-890.
de Sousa, M.V., R.M. Pereira, R. Fukui, V.F. Caparbo, and M.E. da Silva (2014). Carbohydrate beverages attenuate bone resorption markers in elite runners. Metabolism 63:1536-1541.
De Souza, M.J., A. Nattiv, E. Joy, M. Misra, N.I. Williams, R.J. Mallinson, J.C. Gibbs, M. Olmsted, M. Goolsby, and G. Matheson (2014). Female athlete triad coalition consensus statement on treatment and return to play of the female athlete triad: 1st International Conference held in San Francisco, California, May 2012 and 2nd International Conference held in Indianapolis, Indiana, May 2013. Br. J. Sports Med. 48:289.
Dolan, E., and C. Sale (2018). Protein and bone health across the lifespan. Proc. Nutr. Soc. 78: 45-55.
Fenton, T.R., M. Eliasziw, A.W. Lyon, S.C. Tough, and D.A. Hanley (2008). Meta-analysis of the quantity of calcium excretion associated with the net acid excretion of the modern diet under the acid ash diet hypothesis. Am. J. Clin Nutr. 88:1159–1166.
Fredericson, M., K. Chew, J. Ngo, T. Cleek, J. Kiratli, and K. Cobb (2007). Regional bone mineral density in male athletes: a comparison of soccer players, runners and controls. Br. J. Sports Med. 41: 664-668.
Haakonssen, E.C., M.L. Ross, E.J. Knight, L.E. Cato, A. Nana, A.E. Wluka, F.M. Cicuttini, B.H. Wang, D.G. Jenkins, and L.M. Burke (2015). The effects of a calcium-rich pre-exercise meal on biomarkers of calcium homeostasis in competitive female cyclists: a randomised crossover trial. PLoS ONE 10(5):e0123302.
Hammond, K.M., C. Sale, W. Fraser, J. Tang, S.O. Shepherd, J.A. Strauss, G.L. Close, M. Cocks, J. Louis, J. Pugh, C. Stewart, A.P. Sharples, and J.P. Morton (2019). Post-exercise carbohydrate and energy availability induce independent effects on skeletal muscle cell signalling and bone turnover: implications for training adaptation. J Physiol. 597:4779-4796.
Hernlund, E., A. Svedbom, M. Ivergård, J. Compston, C. Cooper, J. Stenmark, E.V. McCloskey, B. Jönsson, and J.A. Kanis (2013). Osteoporosis in the European Union: medical management, epidemiology and economic burden. A report prepared in collaboration with the International Osteoporosis Foundation (IOF) and the European Federation of Pharmaceutical Industry Associations (EFPIA). Arch. Osteoporos. 8:136.
Holick, M.F. (2007). Vitamin D deficiency. N. Engl. J. Med. 357:266-281.
Ihle, R., and A.B. Loucks (2004). Dose-response relationships between energy availability and bone turnover in young exercising women. J. Bone Miner. Res. 19:1231-1240.
Institute of Medicine. Dietary Reference Intakes for Calcium and Vitamin D: Institute of Medicine of the National Academies, 2010.
Kerstetter, J.E., K.O. O’Brien, D.M. Caseria, D.E. Wall, and K.L. Insogna (2005). The impact of dietary protein on calcium absorption and kinetic measures of bone turnover in women. J. Clin. Endocrinol. Metab. 90:26-31.
Kohrt, W.M., P. Wolfe, V.D. Sherk, S.J. Wherry, T. Wellington, E.L. Melanson, C.M. Swanson, C.M. Weaver, and R.S. Boxer (2019). Dermal calcium loss is not the primary determinant of parathyroid hormone secretion during exercise. Med. Sci. Sports Exerc. 51:2117-2124.
Larson-Meyer, D.E., K. Woolf, and L. Burke (2018). Assessment of nutrient status in athletes and the need for supplementation. Int. J. Sports Nutr. Exerc. Metab. 28:139-158.
Macdonald, H.M., S.A. New, W.D. Fraser, M.K. Campbell, and D.M. Reid (2005). Low dietary potassium intakes and high dietary estimates of net endogenous acid production are associated with low bone mineral density in premenopausal women and increased markers of bone resorption in postmenopausal women. Am. J. Clin. Nutr. 81:923–933.
McBryde, A.M. (1985). Stress fractures in runners. Clin. Sports Med. 4:737-752.
Mitchell, P.J., C. Cooper, B. Dawson-Hughes, C.M. Gordon, and R. Rizzoli (2015). Life-course approach to nutrition. Osteoporos. Int. 26:2723-2742.
Moran, D.S., Y. Heled, Y. Arbel, E. Israeli, A.S. Finestone, R.K. Evans, and R. Yanovich (2012). Dietary intake and stress fractures among elite male combat recruits. J Int Soc Sports Nutr. 9(1), 6.
Morton, R.W., K.T. Murphy, S.R. McKellar, B.J. Schoenfeld, M. Henselmans, E. Helms, A.A. Aragon, M.C. Devries, L. Banfield, J.W. Krieger, and S.M. Phillips (2018). A systematic review, meta-analysis and meta-regression of the effect of protein supplementation on resistance training-induced gains in muscle mass and strength in healthy adults. Br. J. Sports Med. 52:376–384.
Mountjoy, M., J. Sundgot-Borgen, L. Burke, S. Carter, N. Constantini, C. Lebrun, N. Meyer, R. Sherman, K. Steffen, R. Budgett, and A. Ljungqvist (2014). The IOC consensus statement: beyond the female athlete triad--relative energy deficiency in sport (RED-S). Br. J. Sports Med. 48:491-497.
Mountjoy, M., J.K. Sundgot-Borgen, L.M. Burke, K.E. Ackerman, C. Blauwet, N. Constantini, C. Lebrun, B. Lundy, A.K. Melin, N.L. Meyer, R.T. Sherman, A.S. Tenforde, M. Klungland Torstveit, and R. Budgett. (2018). IOC consensus statement on relative energy deficiency in sport (RED-S): 2018 update. Br. J. Sports Med. 52:687-697.
National Institute for Health and Clinical Excellence. Osteoporosis fragility fracture risk - Costing report. 2012.
Nattiv, A., A.B. Loucks, M.M. Manore, C.F. Sanborn, J. Sundgot-Borgen, and M.P. Warren (2007). American College of Sports Medicine position stand. The female athlete triad. Med. Sci. Sports Exerc. 39:1867-1882.
Owens, D.J., W.D. Fraser, and G.L. Close (2015). Vitamin D and the athlete: emerging insights. Eur. J. Sport Sci. 15:73-84.
Palacios, C. (2006). The role of nutrients in bone health, from A to Z. Crit. Rev. Food Sci. Nutr. 46: 621-628.
Papageorgiou, M., K.J. Elliott-Sale, A. Parsons, J.C.Y. Tang, J.P. Greeves, W.D. Fraser, and C. Sale (2017). Effects of reduced energy availability on bone metabolism in women and men. Bone 105:191-199.
Ranson, C.A., A.F. Burnett, and R.W. Kerslake (2010). Injuries to the lower back in elite fast bowlers: acute stress changes on MRI predict stress fracture. J. Bone Joint Surg. Br. 92:1664-1668.
Sale, C., I. Varley, T.W. Jones, R.M. James, J.C. Tang, W.D. Fraser, and J.P. Greeves (2015). Effect of carbohydrate feeding on the bone metabolic response to running. J. Appl. Physiol. 119:824-830.
Scientific Advisory Committee on Nutrition. Vitamin D and Health. 2016; https://www.gov.uk/government/groups/scientific-advisory-committee-on-nutrition.
Tenforde, A.S., M.T. Barrack, A. Nattiv, and M. Fredericson (2016). Parallels with the female athlete triad in male athletes. Sports Med. 46:171-182.
Walsh, J.S., and D.B. Henriksen (2010). Feeding and bone. Arch. Biochem. Biophys. 503:11-19.
Zimmermann, E.A., B. Busse, and R.O. Ritchie (2015). The fracture mechanics of human bone: influence of disease and treatment. Bonekey Rep. 4:743.