KEY POINTS
- The ketone bodies acetoacetate (AcAc) and β-hydroxybutyrate (βHB) have wide-ranging metabolic and molecular effects on organs such as the brain, heart, and skeletal muscle, some of which are suggestive of potential benefits to athletes in terms of performance and recovery.
- The recent development and increasing commercial availability of ingestible forms of ketone bodies as exogenous ketone supplements have amplified interest in these products and resulted in many human exercise studies in the past five years.
- This class of supplements currently comprised of ketone salts, ketone esters, and ketogenic compounds such as 1,3-butanediol (BD) and medium chain triglycerides (MCTs), all of which produce, to varying magnitudes and time courses, an acute transient increase in circulating KB concentrations.
- While there are mechanistic bases for potential beneficial effects of exogenous ketone supplements in various athletic contexts, most studies to date have failed to observe benefits to performance or recovery.
- Future research should investigate whether there are other athletic contexts in which exogenous ketone supplements are efficacious given the positive, albeit preliminary, data from studies on overreaching, acute hypoxic exposure, and traumatic brain injury.
INTRODUCTION
For the best part of a century onwards from the late 19th century, ketone bodies (KBs) were associated with the negative consequences of uncontrolled diabetes due to the overproduction of KBs being largely responsible for the clinical manifestation of the life-threatening state of diabetic ketoacidosis (VanItallie & Nufert, 2003). In contrast, the therapeutic value of increased circulating KB concentrations in nonpathological states achieved through either diet-induced hyperketonemia and/or acute nutritional ketosis is increasingly recognized (Poff et al., 2020; VanItallie & Nufert, 2003). These KBs, namely acetoacetate (AcAc), β-hydroxybutyrate (βHB) and acetone, are lipid-derived, water soluble organic compounds produced almost exclusively in the liver, and whose production is amplified most obviously during physiological states characterized by low carbohydrate (CHO) availability i.e. starvation, prolonged fasting, or undertaking ketogenic diets (Poff et al., 2020; Robinson & Williamson, 1980).
AcAc and βHB have pleiotropic effects in multiple organs including the brain, heart, and skeletal muscle by serving as an alternative substrate for energy provision (Robinson & Williamson, 1980), such that there has been a long-standing interest in the development of ingestible forms of KBs for therapeutic purposes (Hashim & VanItallie, 2014). Yet only recently (circa 2015) have commercially available exogenous ketone supplements (EKS) emerged (Evans et al., 2022; Poff et al., 2020). This class of supplements is currently comprised of ketone salts, ketone esters, and ketogenic compounds such as 1,3-butanediol (BD) and medium chain triglycerides (MCTs), all of which produce, to varying magnitudes and time courses, an acute transient increase in circulating KB concentrations (Table 1). This increase has been termed “acute nutritional ketosis” or “intermittent exogenous ketosis” when circulating βHB concentrations are ≥ 0.5 mM (Evans et al., 2022; Poff et al., 2020).
Recent studies have identified other pleiotropic effects of KBs including modulating inflammation, oxidative stress, catabolic processes, and gene expression (Puchalska & Crawford, 2021). In addition, of particular relevance to athletic contexts, are the well-established metabolic actions of KBs to alter substrate utilization through attenuating glucose utilization in peripheral tissues, anti-lipolytic effects on adipose tissue, and attenuating proteolysis in skeletal muscle (Evans et al., 2017; Robinson & Williamson, 1980). With the use of EKS in professional cycling apparently widespread (Cary, 2018; VeloNews, 2020), and the global market for EKS is projected to reach ~US $650 million by 2027 with a compound annual growth rate of ~5.1% during this period (ResearchAndMarkets.com, 2021), there is considerable interest in EKS and their application in athletic contexts. The purpose of this Sports Science Exchange article is therefore to describe the types of EKS currently available, the mechanistic bases for the potentially beneficial (ergogenic) and detrimental (ergolytic) effects of EKS, and to briefly summarize the findings to date for effects on exercise performance and recovery outcomes relevant to athletes.
TYPES OF EXOGENOUS KETONE SUPPLEMENTS AND THEIR EFFECT ON CIRCULATING KETONE BODY CONCENTRATIONS
Circulating KB concentrations are typically ≤ 0.1 mM in the fed state and ~0.2 or ~0.3 mM after an overnight fast. Circulating concentrations may reach ~1.0 mM and ~5.0 mM after 24 h and 1 week of fasting respectively, and ~0.5 to 3.0 mM on a ketogenic diet. Ingestion of EKS is undertaken with the aim of elevating circulating βHB concentrations, and this effect can occur within minutes of ingestion and be maintained for several hours depending on the type and dose of EKS, while also being influenced by factors including being fasted or fed, and being at rest or exercising, such that large variations exist in the degree of ketosis produced (Evans et al., 2022). The various types of EKS that are currently commercially available and/or have been employed in human conditions and their effect on circulating KB concentrations are summarized in Table 1.
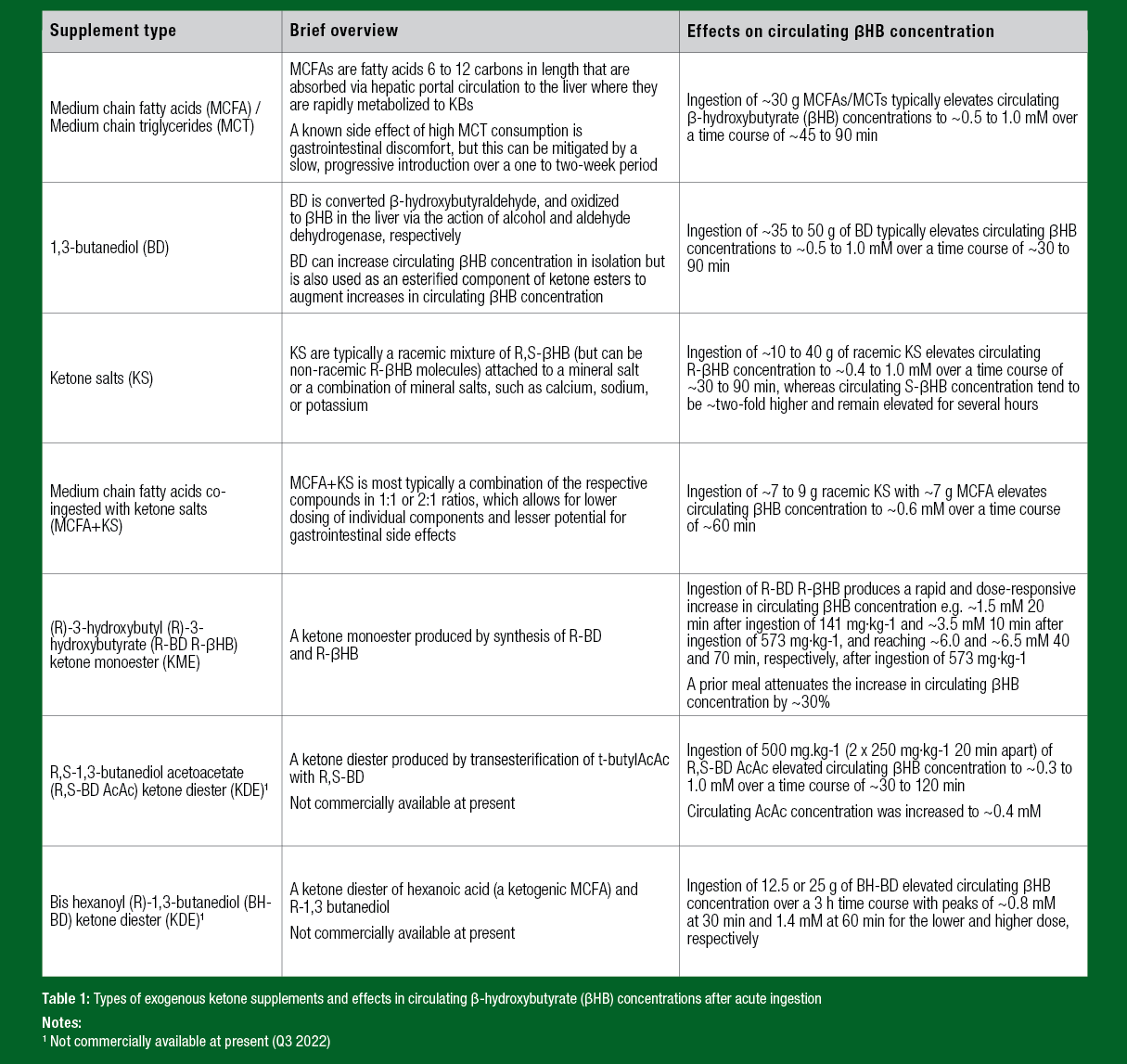
The most direct method of exogenously inducing ketosis would be to ingest isolated KBs. However, βHB and AcAc in their free acid form can be unstable, expensive, and ineffective at producing sustained ketosis. Thus, the ketone acids can be buffered with sodium or other electrolytes to enhance efficacy and prevent overload of any single mineral. There are two enantiomers of βHB, namely R-βHB and S-βHB (also known as D- and L-, respectively). R-βHB is the primary form of βHB in the circulation under physiological conditions, but S-βHB concentrations can be elevated with the consumption of EKS that comprise the R-βHB and S-βHB enantiomers, which is often the case with “racemic” ketone salts (i.e. R,S-βHB salts). Currently, most commercially available ingestible ketone salts are a racemic mixture of R-βHB and S-βHB enantiomers, largely because the synthesis of racemic mixtures is more affordable than the pure enantiomers.
Medium chain triglycerides/fatty acids (MCT/MCFA) and BD are ketogenic precursors and therefore included under the broad category of EKS by virtue of their effects on increasing circulating βHB concentrations after acute ingestion. MCT/MCFA or BD can each be ingested alone, or used in combination with KBs, typically in the form of MCFA+ketone salt formulations, or in the case of BD as a backbone in the synthesis of the ketone esters (Table 1).
Ketone esters is a term used to describe R-βHB and AcAc molecules attached either to another KB or a KB precursor via an ester bond, which is then cleaved by gastric esterases to liberate KBs in their free acid form from a backbone molecule such as R-BD or R,S-BD. Ketone esters used in the exercise science literature include the R-3-hydroxybutyl R-3-hydroxybutyrate (R-BD R-βHB) ketone monoester (KME) (Cox et al., 2016), originally developed to improve the physical and cognitive performance in warfighters (Ford &Glymour, 2014), and the R,S-1,3-butanediol acetoacetate (R,S-BD AcAc) ketone diester (KDE) (Leckey et al., 2017). A recent addition to the ketone ester category is a diester of hexanoic acid (a ketogenic MCFA) and R-1,3 butanediol (BHBD) (Crabtree et al., 2022). Given the numerous possible combinations of AcAc and βHB with ketogenic precursors (including BD, MCFAs, glycerol, and ketogenic amino acids), it is likely that additional forms of EKS will be developed in the future.
At tolerable doses in humans, acute ingestion of MCTs, BD, ketone salts, and the R,S-BD AcAc KDE typically elevate circulating βHB concentrations by ~0.3 to 1.0 mM above resting values, whereas acute ingestion of the R-BD R-βHB KME typically elevates circulating βHB concentrations into the ~3 to 6 mM range, and concentrations during exercise in the ~1.5 to 4.0 mM range (Evans et al., 2022). Ketone esters, especially the R-BD R-βHB KME, are therefore currently the most potent EKS available in terms of reliably and robustly producing acute nutritional ketosis (Table 1). These differences between types of EKS and their effect on circulating βHB concentrations are salient because concentration-dependent effects of βHB and AcAc are likely to exist. As a result, a threshold that acute nutritional ketosis must exceed before effects on skeletal muscle metabolism and exercise performance are observed has been proposed as >1.0 mM (Evans et al., 2017; Shaw et al., 2020).
WHY IS THERE INTEREST IN EXOGENOUS KETONE SUPPLEMENTS AS ERGOGENIC AIDS?
The main physiological role of the amplification of ketogenesis during low CHO availability is for KBs to replace glucose as the primary source of fuel for the brain, and to a lesser extent provide an additional substrate for other peripheral tissues such as heart and skeletal muscle (Puchalska & Crawford, 2021; Robinson & Williamson, 1980). The metabolic consequences of acute nutritional ketosis are such that there are three somewhat inter-related mechanisms by which EKS could potentially act as ergogenic aids, namely (i) the oxidation of KBs as an alternative substrate in contracting skeletal muscle, (ii) better efficiency of ATP production when KBs are used as a substrate during exercise, and (iii) an effect of KBs to elicit reduced reliance on CHO utilization during exercise (Table 2). Based on the available data garnered after ingestion of the R-BD R-βHB KME, oxidation of KBs during exercise makes only a minor (~5%) contribution to ATP provision (Dearlove et al., 2021a; b), and while there is one report of improved delta efficiency during cycling (Dearlove et al., 2021a), the evidence for reduced reliance on CHO utilization is equivocal (Cox et al., 2016; Poffé et al., 2020). These mechanisms, therefore, remain largely unexplored, and in the absence of performance benefits as described below, the ergogenic potential of acute ingestion of EKS is speculative at present. Conversely, there are also several mechanisms by which EKS could potentially have ergolytic effects on exercise performance that also relate to altered patterns of substrate utilization as well as gastrointestinal considerations (Table 2).
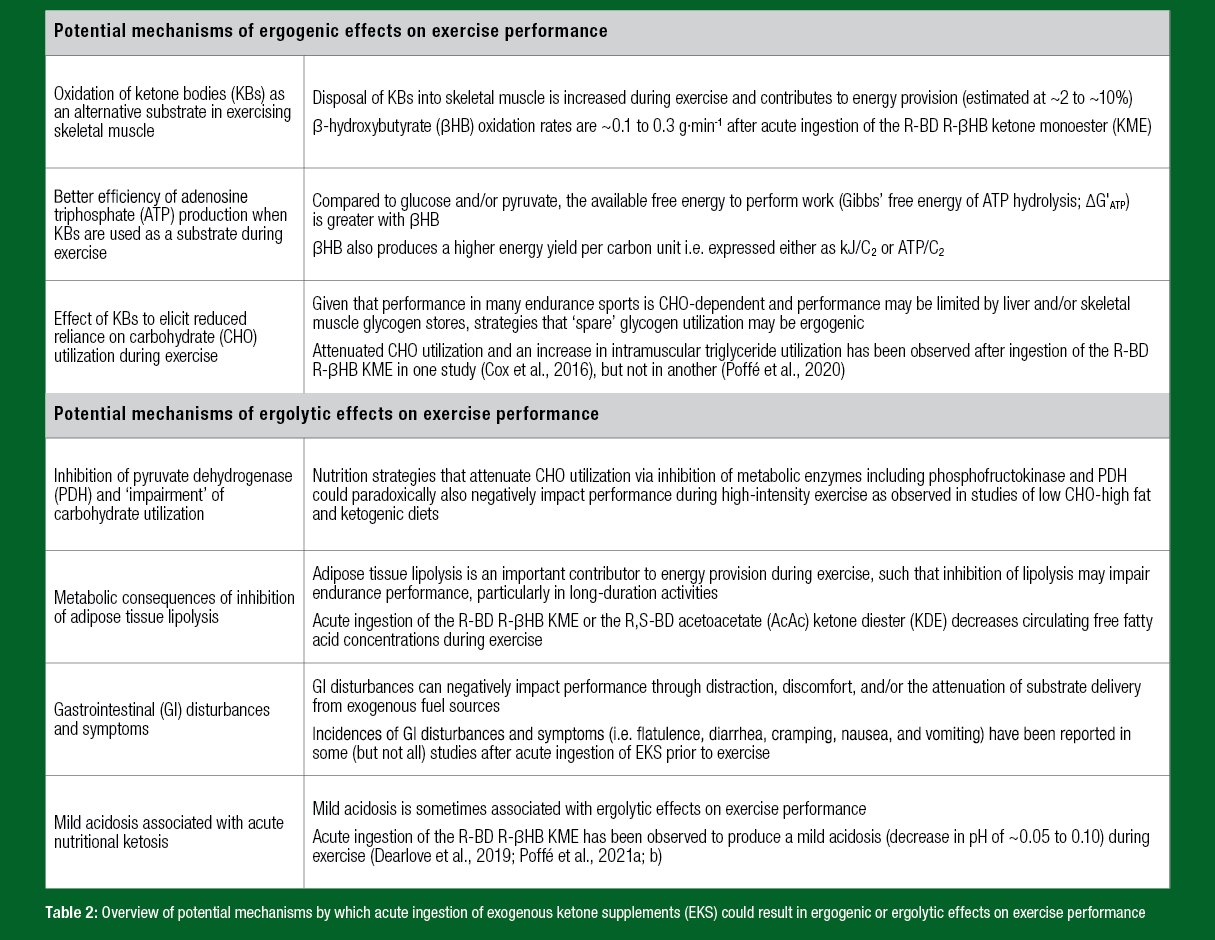
EFFECTS OF ACUTE INGESTION OF EXOGENOUS KETONE SUPPLEMENTS ON EXERCISE PERFORMANCE
The first peer-reviewed article detailing the effects of acute ingestion of EKS in humans on exercise metabolism and endurance performance was published in 2016 (Cox et al., 2016). Ingestion of 573 mg·kg-1 of the R-BD R-βHB KME prior to exercise increased circulating βHB concentrations to ~2.0 to 2.5 mM during exercise, and improved maximum distance in a 30 min cycling time trial (TT) by ~2% (411 ± 162 m; mean ± SEM) when performed following a 1 h pre-load at 75%Wmax (Cox et al., 2016). Since this landmark paper, there has been a dramatic increase in the number of articles investigating the effects in humans of acute ingestion of EKS of various types on exercise metabolism and performance (reviewed in Evans et al., 2022; Shaw et al., 2020; Valenzuela et al., 2021). The next sections briefly summarize these findings.
Ketone Salts
Studies on the effects of acute ingestion of ketone salts on exercise performance have generally provided ~7 to 30 g of R,S-βHB, and have been consistent in producing only modest increases in circulating βHB concentrations (generally ~0.5 to 1.0 mM) with the dosing strategies employed to date (Evans et al., 2022; Valenzuela et al., 2021). Exercise performance in these studies has been largely unchanged in the ketone salts conditions (Evans et al., 2022; Valenzuela et al., 2021). However, one study observed impaired performance after ingestion of 0.3 g·kg-1 of ketone salts (~25 g R,S-βHB) 50 min prior to exercise, as evidenced by a ~7% lower average power output (-16 W) during a 150 kJ cycling TT (~10 km) (O'Malley et al., 2017). In contrast, an ergogenic effect of acute ingestion of ketone salts on exercise performance has been observed in two studies (Kackley et al., 2020; Quinones & Lemon, 2022), but the results are confounded by the fact that the ketone salts condition provided a multi-ingredient pre-workout formulation containing ~100 to 120 mg of caffeine, ~2.8 g of L-taurine, and ~2.1 g of L-leucine in addition to ~7.2 g of R,S-βHB. Performance after ingestion of a caffeine-free version of this formulation did not differ from ingestion of an isoenergetic CHO placebo, which suggests that the presence of caffeine is likely to be a strong influence of the ergogenic effect of that pre-workout formulation (Quinones & Lemon, 2022). As a whole, there is little evidence for ergogenic effects of acute ingestion of ketone salts that are currently commercially available, although one caveat is that most of the performance outcomes have focused on tests of short duration, high-intensity exercise performance (Evans et al., 2022).
Ketogenic Precursors: Medium Chain Triglycerides (MCTs) and 1,3-Butanediol (BD)
MCTs were investigated initially as a means to increase circulating MCFA concentrations, rather than circulating KB concentrations, and thereby to provide an additional energy source during exercise when oxidation rates of orally-ingested glucose reached a maximum at ~1.2 g·min-1. However, the effects of MCT/MCFA ingestion on exercise performance are equivocal (Clegg, 2010). The most recent interest in MCFAs is as ketogenic precursors in combination with ketone salts to elicit higher circulating βHB concentrations than either compound alone.
To date, two studies have investigated the metabolic and performance effects of simultaneous MCFA and ketone salt ingestion in humans (Prins et al., 2020a; b). Both studies employed the same design of ingestion 60 min prior to performing a 5 km treadmill-based running TT, with the MCFA+KS supplement containing ~7 g MCFAs and ~7 to 9 g R,S-βHB per serving, consumed as a single serving (Prins et al., 2020a; b), or double serving (Prins et al., 2020a). Circulating βHB concentrations increased to ~0.6 to 0.7 mM 60 min after ingestion, but neither study observed an effect on the primary outcome of time to complete the 5 km TT when compared to a flavor-matched non-caloric placebo. To date, two studies have investigated the effect of acute pre-exercise ingestion of BD on subsequent endurance performance (Scott et al., 2019; Shaw et al., 2019). The first study employed a 60 min pre-load of submaximal running followed by a 5 km treadmill-based running TT (Scott et al., 2019), whereas the second study employed a 7 kJ·kg-1 cycling TT (Shaw et al., 2019), and neither observed ergogenic effects of BD ingestion. In both studies, circulating βHB concentrations averaged below 1 mM throughout the exercise period, whether after ingestion of 0.5 g·kg-1 BD (Scott et al., 2019), or 2 x 0.35 g·kg-1 BD (Shaw et al., 2019). Therefore, the low-level perceptual (euphoria, dizziness) and gastrointestinal symptoms (low-level nausea, belching and burping), coupled with this modest effect on circulating βHB concentrations suggests that BD is unlikely to have ergogenic potential in athletes.
Ketone Esters
Since the original observation of an improvement in cycling TT performance by ~2% after ingestion of the R-BD R-βHB KME (Cox et al., 2016), many studies examining the effects of acute ingestion of ketone esters on exercise performance have been reported (Dearlove et al., 2019; Evans & Egan, 2018; Evans et al., 2019; Leckey et al., 2017; McCarthy et al., 2021; Peacock et al., 2022; Poffé et al., 2020; 2021a; b; c; Waldman et al., 2022) (Table 3). Only one of these studies investigated ingestion of the R,S-BD AcAc KDE alongside a CHObased fueling strategy, and observed a 2 ± 1% (58.2 s) decrement in 31.2 km TT performance, and 3.7% reduction in average power output, in professional cyclists (Leckey et al., 2017). Circulating βHB concentrations were ~0.4 mM and therefore markedly lower than achieved with R-BD R-βHB KME ingestion, and were accompanied by a high prevalence of gastrointestinal symptoms not present with CHO alone (Leckey et al., 2017).
The remaining studies using ketone esters have investigated acute ingestion of the R-BD R-βHB KME. Most of these studies have observed negligible effects on exercise performance (Table 3). However, one study observed an ergogenic effect in professional rugby union players performing a simulated rugby union-specific match-play protocol (Peacock et al., 2022). Compared to CHO alone, ingestion of 590 mg·kg-1 of the R-BD R-βHB KME with CHO before and during exercise produced circulating βHB concentrations of > 2.0 mM and resulted in a 0.33 ± 0.41 s (2.1%) improvement in the average time to complete a sustained high-intensity performance test performed regularly throughout the protocol. However, 15 m sprint times and sled push performance during the protocol were not different between conditions (Peacock et al., 2022). In contrast, performance in a 30 min TT (preceded by 60 min pre-load of intermittent intensity cycling exercise) was impaired by ~1.5% in trained cyclists after ingestion of 50 g of the R-BD R-βHB KME alongside a CHO-based fueling strategy that had resulted in circulating βHB concentrations of ~3.5 mM throughout the TT (Poffé et al., 2021c).
Recent reviews of the field unsurprisingly concluded that at present there is a lack of evidence for the ergogenic effect of ketone esters (Evans et al., 2022; Valenzuela et al., 2021). Yet given the variety of factors that can influence the efficacy of dietary supplements in performance contexts, e.g. the specifics of the performance test, the training level of participants, the fed/fasted status, and nutrient congestion prior to and during performance, and specific to EKS, the circulating βHB concentration reached during exercise, the possibility remains that there are specific performance contexts where acute ingestion of ketone esters would provide an ergogenic effect.
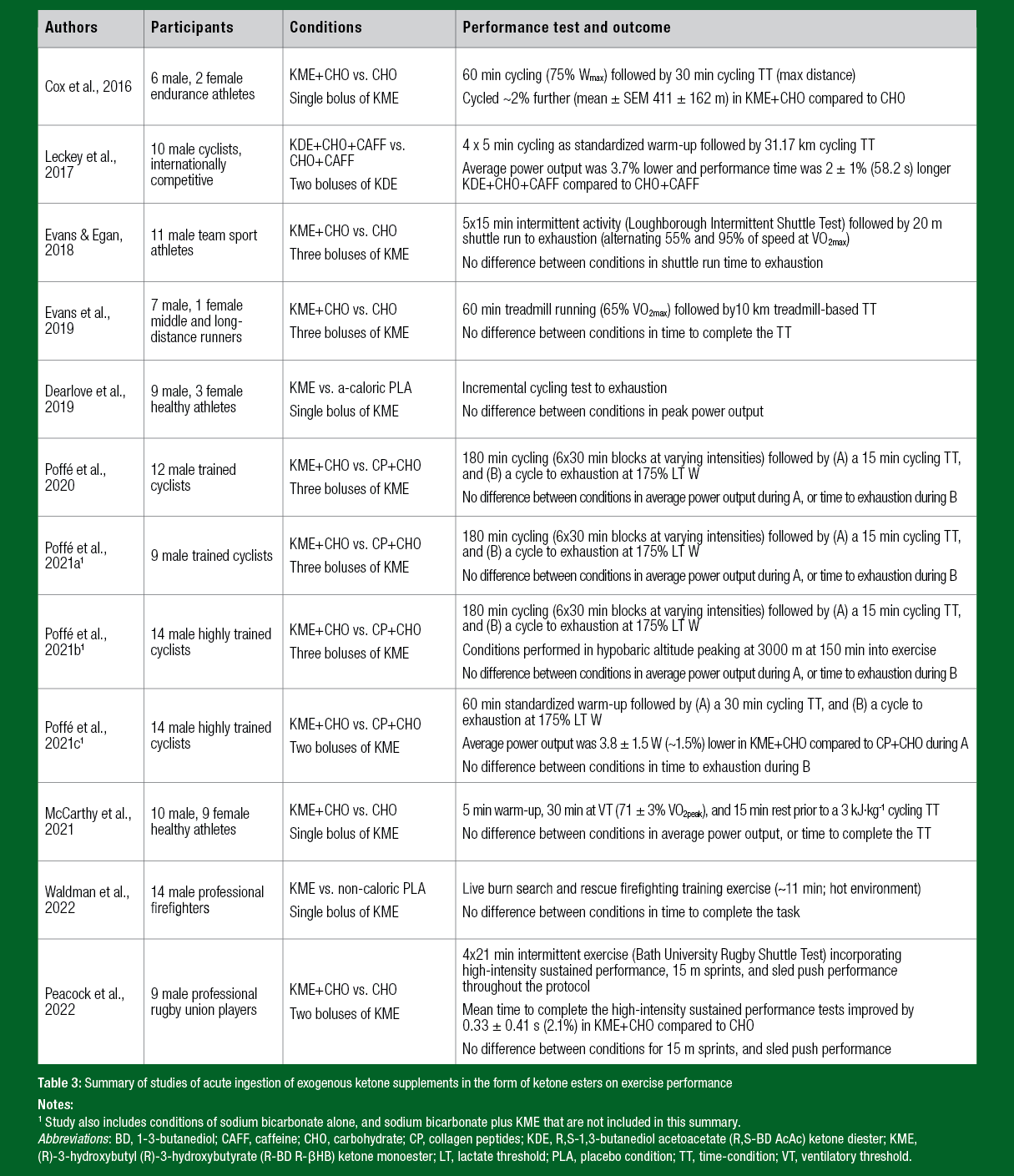
WHERE TO NEXT FOR EXOGENOUS KETONE SUPPLEMENTS IN ATHLETIC CONTEXTS?
The apparent popularity of EKS in elite professional cycling (Cary, 2018; Velo News, 2020) is difficult to reconcile with the lack of evidence for ergogenic effects in the published literature. Because laboratory based studies of dietary supplements rarely study elite athletes in ecologically-valid performance contexts, nor do currently-published studies faithfully mimic the duration and context of professional cycling in three-week Grand Tours, it may be that performance benefits that are obvious to these teams and athletes are not being captured by laboratory-based studies of EKS to date. Further studies may be warranted to investigate whether there are optimal dosing strategies, specific athletic populations, and/or specific exercise challenges, in which acute ingestion of EKS may provide an ergogenic benefit. Other potential avenues of interest include augmenting recovery from acute exercise, and mitigation of the deleterious effects of acute hypoxic exposure, traumatic brain injury, and catabolic states.
Recovery From Acute Exercise
Acute ingestion of EKS has been investigated as a method to complement
existing best-practice CHO and protein-based nutrition strategies and augment glycogen resynthesis and muscle protein synthesis (MPS) during recovery, but effects are equivocal. One study investigated the ingestion of 573 mg·kg-1 of the R-BD R-βHB KME alongside a 2 h 10 mM hyperglycaemic clamp performed immediately after a glycogendepleting bout of exercise, and reported absolute muscle glycogen concentrations being ~50% greater compared to the control condition (Holdsworth et al., 2017). However, the difference between conditions expressed as relative change in muscle glycogen concentration compared to respective post-exercise values was modest. Another study of post-exercise ingestion of the R-BD R-βHB KME combined with hourly recovery drinks providing 1.0 g·kg-1.h-1 CHO and 0.3 g·kg- 1·h-1 whey protein hydrolysate observed no effect on muscle glycogen resynthesis during a 5 h recovery period after 90 min of unilateral leg extension exercise (Vandoorne et al., 2017). One notable secondary outcome was the observation with R-BD R-βHB KME ingestion of greater phosphorylation of S6K1 and 4E-BP1 during recovery, which are two key regulators of MPS via the canonical mechanistic target of rapamycin (mTOR) pathway.
Acute Hypoxic Exposure
Alternative fueling strategies that attenuate declines in systemic and skeletal muscle oxygenation, exercise performance, and symptoms of mountain sickness, may provide ergogenic benefits during moderate to- high altitude exposure. Acute ingestion of the R-BD R-βHB KME can increase systemic and skeletal muscle oxygenation at rest and during exercise with hypoxic exposure (Coleman et al., 2021; Poffé et al., 2021b), whereas at rest, the decline in cognitive performance during severe hypoxic exposure (simulating an altitude of ~5000 m) was attenuated by acute nutritional ketosis (Coleman et al., 2021). Traumatic Brain Injury Traumatic brain injury (TBI) can occur in athletic contexts, with the severity ranging from small but repeated sub-concussive insults up to overt high-impact insult resulting in loss of consciousness. Ketosis achieved by ketogenic diet or ingestion of EKS has been widely hypothesized to present a promising prophylactic and/or post-injury therapeutic strategy for TBI through a mechanism whereby KBs serve as an alternative substrate for energy provision during the post-injury period of impaired glycolytic metabolism. Ultimately, glucose becomes a less favorable energy substrate and ATP provision decreases, whereas KBs are an alternative substrate that can contribute significantly to cerebral metabolism (Daines, 2021; Stubbs et al., 2021). Recently, postinjury daily oral gavage of the R-BD R-βHB KME protected against TBIinduced morphological and functional deficits in Sprague-Dawley rats subjected to a controlled cortical impact (Almeida-Suhett et al., 2022).
Catabolic States
Anti-catabolic effects of KBs (Koutnik et al., 2019), coupled with the potential anabolic effects of acute nutritional ketosis, i.e. a 10% increase in MPS during 6 h infusion of sodium R,S-βHB (Nair et al., 1988), suggest that EKS may support maintenance of skeletal muscle mass in high-stress environments (Stubbs et al., 2021). Of particular relevance to athletes would be scenarios where athletes are at risk for excessive protein breakdown, inadequate recovery, and overreaching, such as during intensified training, phases of intentional weight loss via energy restriction such as in weight category sports, and periods of forced inactivity due to injury.
One study suggested that the daily consumption of the R-BD R-βHB KME (75 g·d-1 as 3 x 25 g) blunted the symptoms of overreaching during three weeks of intensive cycling-based training in physically active young men (Poffé et al., 2019). One confounder in this study was an important between-group difference that arose in the dietary intake data, as a ‘spontaneous’ ~20% increase in energy intake was observed in the KME group (Poffé et al., 2019). While several performance measures showed no between-condition differences during the study, power output during a 30 min cycling TT performed after a 90 min preload was ~15% greater with R-BD R-βHB KME compared to control on day 18 of training (Poffé et al., 2019).
PRACTICAL APPLICATIONS
- A working hypothesis remains that there is a threshold (likely to be > 1.0 mM) above which βHB concentrations must exceed in order to elicit acute metabolic effects relevant to exercise performance.
- The presently high cost and/or limited availability of ketone esters, and the negative consequences of either intoxication like effects of BD or high mineral load with ketone salts, are barriers to be overcome if EKS are to be widely used in sport.
- Presently, the majority of the available evidence does not provide support for the acute ingestion of EKS producing direct performance benefits in athletic contexts.
- Questions remain about whether there are optimal dosing strategies (especially using ketone esters), specific athletic populations, and/or specific exercise challenges, by which acute ingestion of EKS may provide an ergogenic benefit.
SUMMARY
There is little doubt that AcAc and R-βHB have wide-ranging metabolic and molecular effects, and that the commercial availability of ingestible EKS has led to the recent interest in KBs in athletic and therapeutic contexts. Despite mechanistic bases for potential beneficial effects of EKS, these putative mechanisms are largely unexplored and remain somewhat speculative, whereas the evidence at present is overwhelmingly against EKS being ergogenic aids for athletic performance. Many nodes of metabolic regulation influencing substrate utilization in exercising skeletal muscle are potentially altered by acute nutritional ketosis, and therefore further investigation is needed to explore the regulation of substrate utilization during exercise after ingestion of EKS, and whether ergogenic or ergolytic effects are more consistently observed. Overall, the data on recovery and overreaching, acute hypoxic exposure, and traumatic brain injury are preliminary but suggest that there may be efficacy for EKS in these contexts. The views expressed are those of the authors and do not necessarily reflect the position or policy of PepsiCo, Inc.
REFERENCES
Almeida-Suhett, C., A.M. Namboodiri, K. Clarke, and P.A. Deuster (2022). The ketone ester, 3-hydroxybutyl-3-hydroxybutyrate, attenuates neurobehavioral deficits and improves neuropathology following controlled cortical impact in male rats. Nutr. Neurosci. 25:1287-1299.
Cary T. (2018) Tour de France riders ready to fuel up on ketones – the mysterious energy drink developed at Oxford University. https://www.telegraph.co.uk/cycling/2018/07/05/tour-de-france-riders-ready-fuel-ketones-mysterious-energy/. Accessed 05/07/2018.
Clegg, M.E. (2010). Medium-chain triglycerides are advantageous in promoting weight loss although not beneficial to exercise performance. Int. J. Food Sci. Nutr. 61:653-679.
Coleman, K., J. Phillips, M. Sciarini, B. Stubbs, O. Jackson, and D. Kernagis (2021). A metabolic intervention for improving human cognitive performance during hypoxia. Aerosp. Med. Hum. Perform. 92:556-562.
Cox, P.J., T. Kirk, T. Ashmore, K. Willerton, R. Evans, A. Smith, A.J. Murray, B. Stubbs, J. West, S.W. McLure, M.T. King, M.S. Dodd, C. Holloway, S. Neubauer, S. Drawer, R.L. Veech, J.L. Griffin, and K. Clarke (2016). Nutritional ketosis alters fuel preference and thereby endurance performance in athletes. Cell Metab. 24:256-268.
Crabtree, C.D., T. Blade, P.N. Hyde, A. Buga, M.L. Kackley, T.N. Sapper, O. Panda, S. Roa-Diaz, J.C. Anthony, J.C. Newman, J.S. Volek, and B.J. Stubbs (2022). Bis hexanoyl (R)-1,3-butanediol, a novel ketogenic ester, acutely increases circulating R- and S-ßhydroxybutyrate concentrations in healthy adults. J. Am. Nutr. Assoc. Online ahead of print. PMID: 35512774.
Daines, S.A. (2021). The therapeutic potential and limitations of ketones in traumatic brain injury. Front. Neurol. 12:723148.
Dearlove, D.J., O.K. Faull, E. Rolls, K. Clarke, and P.J. Cox (2019). Nutritional ketoacidosis during incremental exercise in healthy athletes. Front. Physiol. 10:290.
Dearlove, D.J., O.K. Harrison, L. Hodson, A. Jefferson, K. Clarke, and P.J. Cox (2021a). The Effect of Blood Ketone Concentration and Exercise Intensity on Exogenous Ketone Oxidation Rates in Athletes. Med Sci Sports Exerc 53:505-516.
Dearlove D.J., D. Holdsworth, T. Kirk, L. Hodson, E. Charidemou, E. Kvalheim, B. Stubbs, A. Beevers, J.L. Griffin, R. Evans, J. Robertson, K. Clarke, and P.J. Cox (2021b). β-hydroxybutyrate oxidation in exercise is impaired by low-carbohydrate and high-fat availability. Front. Med. 8:721673.
Evans, M., and B. Egan (2018). Intermittent running and cognitive performance after ketone ester ingestion. Med. Sci. Sports Exerc. 50:2330-2338.
Evans, M., K.E. Cogan, and B. Egan (2017). Metabolism of ketone bodies during exercise and training: physiological basis for exogenous supplementation. J. Physiol. 595:2857-2871.
Evans, M., F.T. McSwiney, A.J. Brady, and B. Egan (2019). No benefit of ingestion of a ketone monoester supplement on 10-km running performance. Med. Sci. Sports Exerc. 51:2506-2515.
Evans, M., T.S. McClure, A.P. Koutnik, and B. Egan (2022). Exogenous ketone supplements in athletic contexts: past, present, and future. Sports Med. doi:10.1007/s40279-022-01756-2.
Ford, K., and C. Glymour (2014). The enhanced warfighter. Bull. At. Sci. 70:43-53.
Hashim, S.A., and T.B. VanItallie (2014). Ketone body therapy: from the ketogenic diet to the oral administration of ketone ester. J. Lipid Res. 55:1818-1826.
Holdsworth, D.A., P.J. Cox, T. Kirk, H. Stradling, S.G. Impey, and K. Clarke (2017). A ketone ester drink increases postexercise muscle glycogen synthesis in humans. Med. Sci. Sports Exerc. 49:1789-1795.
Kackley, M.L., J.A. Short, P.N. Hyde, R.A. LaFountain, A. Buga, V.J. Miller, R.M. Dickerson, T.N. Sapper, E.C. Barnhart, D. Krishnan, C.A. McElroy, C.M. Maresh, W.J. Kraemer, and J.S. Volek (2020). A Pre-workout supplement of ketone salts, caffeine, and amino acids improves high-intensity exercise performance in keto-naïve and keto-adapted individuals. J. Am. Coll. Nutr. 39:290-300.
Koutnik, A.P., D.P. D'Agostino, and B. Egan (2019). Anticatabolic effects of ketone bodies in skeletal muscle. Trends Endocrinol. Metab. 30:227-229.
Leckey, J.J., M.L. Ross, M. Quod, J.A. Hawley, and L.M. Burke (2017). Ketone diester ingestion impairs time-trial performance in professional cyclists. Front. Physiol. 8:806.
McCarthy, D.G., W. Bostad, F.J. Powley, J.P. Little, D.L. Richards, and M.J. Gibala (2021). Increased cardiorespiratory stress during submaximal cycling after ketone monoester ingestion in endurance-trained adults. Appl. Physiol. Nutr. Metab. 46:986-993.
Nair, K.S., S.L. Welle, D. Halliday, and R.G. Campbell (1988). Effect of beta-hydroxybutyrate on whole-body leucine kinetics and fractional mixed skeletal muscle protein synthesis in humans. J. Clin. Invest. 82:198-205.
O'Malley, T., E. Myette-Cote, C. Durrer, and J.P. Little (2017). Nutritional ketone salts increase fat oxidation but impair high-intensity exercise performance in healthy adult males. Appl, Physiol, Nutr, Metab, 42:1031-1035.
Peacock, O.J., J.T. Gonzalez, S.P. Roberts, A. Smith, S. Drawer, and K.A. Stokes (2022). Ketone monoester ingestion alters metabolism and simulated rugby performance in professional players. Int. J. Sport Nutr. Exerc. Metab. 32:334-341.
Poff, A.M., A.P. Koutnik, and B. Egan (2020). Nutritional ketosis with ketogenic diets or exogenous ketones: Features, convergence, and divergence. Curr. Sports Med. Rep.19:251-259.
Poffé, C., M. Ramaekers, R. Van Thienen, and P. Hespel (2019). Ketone ester supplementation blunts overreaching symptoms during endurance training overload. J. Physiol. 597:3009-3027.
Poffé, C., M. Ramaekers, S. Bogaerts, and P. Hespel (2020). Exogenous ketosis impacts neither performance nor muscle glycogen breakdown in prolonged endurance exercise. J. Appl. Physiol. 128:1643-1653.
Poffé, C., M. Ramaekers, S. Bogaerts, and P. Hespel (2021a). Bicarbonate unlocks the ergogenic action of ketone monoester intake in endurance exercise. Med. Sci. Sports Exerc. 53:431-441.
Poffé, C., R. Robberechts, T. Podlogar, M. Kusters, T. Debevec, and P. Hespel (2021b). Exogenous ketosis increases blood and muscle oxygenation but not performance during exercise in hypoxia. Am. J. Physiol. 321:R844-R857.
Poffé, C., F. Wyns, M. Ramaekers, and P. Hespel (2021c). Exogenous ketosis impairs 30-min time-trial performance independent of bicarbonate supplementation. Med. Sci. Sports Exerc. 53:1068-1078.
Prins, P.J., D.P. D'Agostino, C.Q. Rogers, D.L. Ault, G.L. Welton, D.W. Jones, S.R. Henson, T.J. Rothfuss, K.G. Aiken, J.L. Hose, E.L. England, A.D. Atwell, J.D. Buxton, and A.P. Koutnik (2020a). Dose response of a novel exogenous ketone supplement on physiological, perceptual and performance parameters. Nutr. Metab. 17:81.
Prins, P.J., A.P. Koutnik, D.P. D'Agostino, C.Q. Rogers, J.F. Seibert, J.A. Breckenridge, D.S. Jackson, E.J. Ryan, J.D. Buxton, and D.L. Ault (2020b). Effects of an exogenous ketone supplement on five-kilometer running performance. J. Hum. Kinet. 72:115-127.
Puchalska, P., and P.A. Crawford (2021). Metabolic and signaling roles of ketone bodies in health and disease. Annu. Rev. Nutr. 41:49-77.
Quinones, M.D., and P.W.R. Lemon (2022). Acute ketone salts-caffeine-taurine-leucine supplementation but not ketone salts-taurine-leucine, improves endurance cycling performance. Int. J. Sport Nutr. Exerc. Metab. 32:238-245.
ResearchAndMarkets.com (2021). Ketones - Global Market Trajectory & Analytics. https://www.researchandmarkets.com/r/pzjjgx. Accessed 02/04/2022 2022
Robinson, A.M., and D.H. Williamson (1980). Physiological roles of ketone bodies as substrates and signals in mammalian tissues. Physiol. Rev. 60:143-187.
Scott, B.E., P.B. Laursen, L.J. James, B. Boxer, Z. Chandler, E. Lam, T. Gascoyne, J.Messenger, and S.A. Mears (2019). The effect of 1,3-butanediol and carbohydrate supplementation on running performance. J. Sci. Med. Sport 22:702-706.
Shaw, D.M., F. Merien, A. Braakhuis, D. Plews, P. Laursen, and D.K. Dulson (2019). The effect of 1,3-butanediol on cycling time-trial performance. Int. J. Sport Nutr. Exerc. Metab. 29:466-473.
Shaw, D.M., F. Merien, A. Braakhuis, E. Maunder, and D.K. Dulson (2020). Exogenous ketone supplementation and keto-adaptation for endurance performance: disentangling the effects of two distinct metabolic states. Sports Med. 50:641-656.
Stubbs, B.J., A.P. Koutnik, J.S. Volek, and J.C. Newman (2021). From bedside to battlefield: intersection of ketone body mechanisms in geroscience with military resilience. Geroscience 43:1071-1081.
Valenzuela, P.L., A. Castillo-García, J.S. Morales, and A. Lucia (2021). Perspective: Ketone supplementation in sports-does it work? Adv. Nutr. 12:305-315.
Vandoorne, T., S. De Smet, M. Ramaekers, R. Van Thienen, K. De Bock, K. Clarke, and P. Hespel (2017). Intake of a ketone ester drink during recovery from exercise promotes mtorc1 signaling but not glycogen resynthesis in human muscle. Front. Physiol. 8:310.
VanItallie, T.B., and T.H. Nufert (2003). Ketones: metabolism's ugly duckling. Nutr. Rev. 61:327-341.
VeloNews (2020) Tour de France leader Primož Roglič confirms Jumbo-Visma’s use of ketones: ‘For the real effects, it’s hard to say’. https://www.velonews.com/events/tourde-france/primoz-roglic-confirms-jumbo-vismas-use-of-ketones-for-the-real-effectsits-hard-to-say/. Accessed 17/09/2020 2020
Waldman, H.S., A.R. Bryant, B.D. Shepherd, B. Egan, and M.J. McAllister (2022). No effect of a ketone monoester on markers of stress and performance in a live-burn search and rescue in firefighters. J. Strength Cond. Res. 36:763-711.