KEY POINTS
- It is well established that moderate to high caffeine doses (5–9 mg/kg body mass (bm)), ingested before and during exercise, increase endurance performance in laboratory and field settings. These doses are associated with increased heart rate and blood catecholamine, lactate, free fatty acid and glycerol levels in many subjects. However, side effects that often occur include gastrointestinal upset, nervousness, mental confusion, inability to focus and disturbed sleep.
- Lower caffeine doses (<3 mg/kg bm ~200 mg) taken before, during and late in exercise also increase endurance performance, and do not cause the physiological changes and side effects noted above in most individuals.
- Caffeine is also ergogenic in many forms of short-term high-intensity exercise and team stop-and-go sports, where anaerobic energy provision plays a significant role in performance success.
- The ergogenic effects of caffeine appear to result from antagonistic interactions with adenosine receptors in the central and peripheral nervous systems, increasing central drive and reducing the perception of effort and pain during exercise.
- The ergogenic effects of caffeine are maintained when administered in alternate forms other than capsules/tablets and coffee, including sports and energy drinks, gels, gum, bars and dissolvable mouth strips. Mouth rinsing with caffeine and aerosol administration is less likely to produce ergogenic effects.
- It is not yet clear whether genetic polymorphisms related to caffeine metabolism or adenosine receptor density can explain the inter-individual variability seen in the ergogenic response to caffeine administration.
INTRODUCTION
Caffeine may be the most studied “supplement” on the planet. It has been shown to be “ergogenic” or “performance-enhancing” in almost every exercise and sporting scenario that has been studied. While caffeine has no nutritional value, it is consumed worldwide in a variety of social and sporting settings and was removed from the restricted list of the World Anti-Doping Agency in 2004. The consumption of caffeine to improve performance dates back many centuries, and published studies examining the effects of caffeine in sport already appeared in the early 20th century (Rivers & Webber, 1907) as reviewed by Burke et al. (2013). However, most people recognize the pioneering work of Dr. David Costill and colleagues at Ball State University in the late 1970s as being responsible for the scientific interest in caffeine. Their work found that trained cyclists improved their cycle times to exhaustion (at ~80% of maximal oxygen consumption (VO2max)), from 75 min in the placebo condition to 96 min following the ingestion of 5 mg/kg body mass (bm) of caffeine (~330 mg) in coffee (Costill et al., 1978). A second study gave 250 mg of caffeine at the beginning of exercise and then another 250 mg in seven doses during exercise and reported a 20% increase in the work completed during 2 h of cycling (Ivy et al., 1979).
Since that time, research has continued to examine many aspects of caffeine consumption in running, cycling, rowing, individual and team sports, resistance exercise, sprinting and many additional exercise or sporting events. The continued interest in caffeine is to the point where “meta analyses” and “review papers” almost outnumber actual “experimental studies” (see Pickering & Grgic, 2019 for a listing), but these articles allow the reader to review the literature regarding the ergogenic effects of caffeine on many types of exercise and sports. The purpose of this Sports Science Exchange article is to examine some of the newer areas of caffeine research and information.
THE CHANGING LANDSCAPE OF CAFFEINE RESEARCH
Caffeine research in exercise and sport settings has changed in the past 10-15 years as there has been interest in, (1) examining the ergogenic effects of low caffeine doses (defined here as <3 mg/kg bm, ~200 mg), including divided doses of caffeine, before and during a wide variety of exercise situations and sports, (2) using real-world exercise tests (e.g., time trials (TT)) and trained athletes to assess caffeine effects on performance during their actual events/sports, (3) administering caffeine in alternate forms, including carbohydrate electrolyte solutions (CES), gels, bars, gums, dissolvable mouth strips, mouth rinsing and aerosols, and (4) the role that genetic variance may play in explaining the variable ergogenic effects of caffeine supplementation.
LOW DOSES OF CAFFEINE ARE ERGOGENIC
Many studies using moderate to high doses of caffeine (5-9 mg/kg bm) reported ergogenic effects in endurance-type activities (Fig. 1A) and pronounced effects on the physiological responses to exercise, including increased heart rate, a doubling of blood catecholamine levels, higher blood lactate levels and also increased blood free fatty acid (FFA) and glycerol levels in some subjects (Graham & Spriet, 1991; 1995; Pasman et al., 1995). These doses also produced some troublesome side effects, including gastrointestinal upset, nervousness, mental confusion, inability to focus and disturbed sleeping. However, the administration of lower caffeine doses (3 mg/kg bm) also produced an ergogenic effect with distinct increases in plasma caffeine concentrations (Fig. 1B), but no changes in the physiological responses to exercise and few, if any, side effects (Desbrow et al., 2012; Graham & Spriet, 1995).
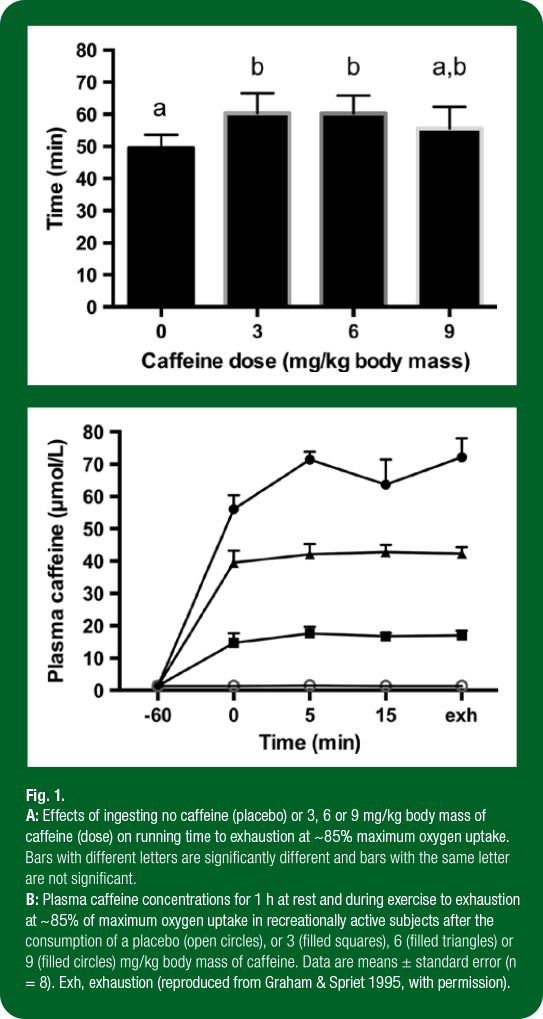
Several recent studies have shown that lower doses of caffeine are ergogenic when given prior to exercise in short and longer endurance events (Clarke et al., 2019; Lane et al., 2014; Pitchford et al., 2014; Skinner et al., 2019), muscle endurance and strength exercises (Grgic et al., 2020a), stop-and-go team sports like basketball, volleyball, soccer, rugby, field hockey (for reviews, see Burke, 2008; Chia et al., 2017; Salinero et al., 2019; Spriet, 2014), and ice hockey (Madden et al., 2019), as well as individual sports like swimming (Lara et al., 2015), golf (Stevenson et al., 2009) and tennis (Gallo-Salazar et al., 2015) in both women and men .
Research has also reported that well-trained athletes are very sensitive to small doses of caffeine late in prolonged exercise without ingesting caffeine before exercise (Cox et al., 2002: Talanian & Spriet, 2016). For example, the effects of two low caffeine doses on TT performance following a prolonged cycle were examined in 15 well-trained women and men cyclists and triathletes, who were not caffeine users (Talanian & Spriet, 2016). They completed four trials in a double-blinded and random fashion where they cycled for 120 min at ~60% VO2max, with five hill climbs at ~85% VO2max, followed by a 6 kJ/kg bm TT lasting 25–30 min. In all trials, subjects consumed 5 mL/kg bm of CES (6 % carbohydrate (CHO), 20 mmol/L sodium) throughout the 120 min. At 80 min, subjects received one of three conditions in their CES: placebo (regular CES), CAF100 (100 mg caffeine, ~1.5 mg/kg bm), CAF200 (200 mg caffeine, ~3 mg/kg bm). The subjects also completed a random “4th trial” (a repeat of one of the three conditions) to establish TT repeatability and help complicate the subjects’ perception of what they had received, with post-trial questionnaires confirming that the “double-blinding” was successful. Subjects were significantly faster in the CAF100 (27:36 ± 0:32 min) trial, and faster again in the CAF200 (26:36 ± 0:22 min) trial vs. placebo (28:41 ± 0:38 min) (Fig. 2). Time-trial performance reliability was good in the “repeat” trials (five subjects completed two placebo trials, five completed two CAF100 trials, and five completed two CAF200 trials) with performance times of 27:19 ± 0:30 and 27:30 ± 0:35 min. Plasma caffeine levels were not measurable in the placebo trial, but reached 14.9 umol/L before (120 min) and 13.8 umol/L after the TT in CAF100, and 24.9 and 25.6 umol/L at the same time points in CAF200. These results demonstrated that low caffeine doses (~1.5 and ~3 mg/kg bm) were ergogenic in a TT in well-trained cyclists when caffeine was ingested late in a 2 h training ride (Talanian & Spriet, 2016). The 200 mg dose caffeine was more potent than 100 mg, but there were no differences in the physiological responses to the initial 120 min of submaximal exercise and prior to the TT between conditions. These results suggested nervous system-based mechanism(s) for the improvement in performance and revealed that very low caffeine doses and plasma levels are ergogenic when the athlete is experiencing some fatigue.
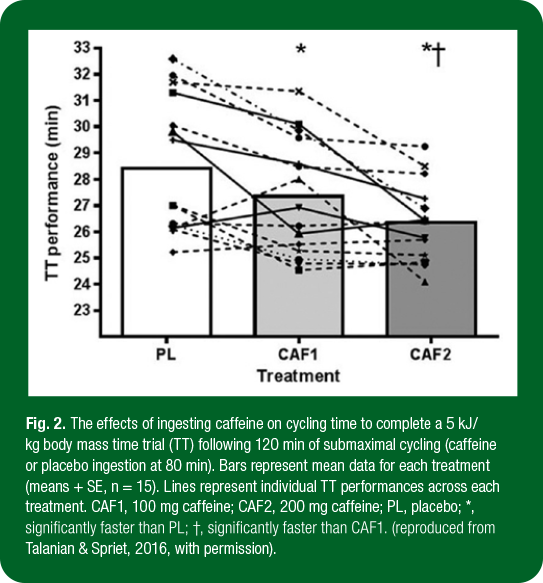
HOW DO LOW CAFFEINE DOSES EXERT AN ERGOGENIC EFFECT?
The initial hypothesis to explain the ergogenic effects of caffeine during endurance exercise was metabolic, with caffeine increasing catecholamine levels and adipose tissue lipolysis, with the resulting increased circulating FFAs taken up and oxidized by the contracting muscle, thereby sparing muscle glycogen stores for use later in exercise, resulting in prolonged work time to exhaustion (Costill et al., 1978). However, increased endurance performance with low doses of caffeine that elicited none of these metabolic changes (Graham & Spriet, 1995) strongly suggested that the ergogenic effect of caffeine was mediated through the central (CNS) and/or peripheral nervous systems (PNS). Previous work demonstrating caffeine’s antagonistic effect on adenosine receptors throughout the body provided the most probable mechanism of action (Fredholm, 1995; Kalmar & Cafarelli, 2004). The most powerful support for a role of the CNS remains the work by Davis et al. (2003) who injected both adenosine agonists and antagonists (caffeine) directly into the brains of rodents (CNS) and demonstrated an ergogenic effect of caffeine on running performance. The mechanisms are related to be antagonism of adenosine receptors by caffeine resulting in increased central drive in the CNS, as well as decreased perception of effort and pain in the PNS, both contributing to improved athletic performance (Bowtell et al., 2018; Kalmar & Cafarelli, 2004). In humans, the difficulty in making invasive measurements in the nervous systems makes it difficult to conduct further research in this area.
ALTERNATE FORMS OF CAFFEINE DELIVERY
The traditional form of caffeine administration in research and athletic settings for a long time was to ingest tablets/capsules along with water or to drink coffee (Hodgson et al., 2013; Clarke et al., 2019). The caffeine is quickly swallowed, and the majority absorbed into the blood from the intestine, with the possibility that a small amount is also absorbed in the buccal mucosa of the oral cavity. Caffeinated sports drinks have also been studied for many years, with most reports demonstrating that caffeine added to a sports drink has a further performance enhancing effect above that of a CES alone (see reviews, Cureton et al., 2007; Spriet, 2014). Caffeine is now also available in gels, bars, gums, dissolvable mouth strips, lozenges and energy drinks, which may affect how quickly the caffeine is absorbed into the blood from the buccal mucosa and intestines. There has also been recent interest in mouth rinsing with caffeine which may activate sensors in the oral cavity with direct connections to the brain that could ultimately affect athletic performance. Lastly, researchers are beginning to examine whether the delivery of caffeine in mouth and nasal aerosols may activate sensors with neural links in the nose and/or provide a direct route for caffeine absorption in the lungs.
Caffeine delivered in chewing gum, bars, gels, mouth strips and energy drinks can be effectively administered at doses up to ~200 mg, and higher with repeated dosing. As might be expected, these forms of delivery are rapidly absorbed into the blood (Kamimori et al., 2002) and result in improvements in athletic performance (Paton et al., 2010; Whalley et al., 2019; Wickham & Spriet, 2018). Studies with caffeinated energy drinks have generally not examined the individual effects of caffeine on performance, as other documented (CHO) and potential (taurine) active ingredients are present. However, when an energy drink with 3 mg/kg bm of caffeine was compared to a caffeine only trial (and matched for CHO intake), the improvement in performance over the placebo trial was similar in the energy drink and caffeine trials (Quinlivan et al., 2015). This strongly suggested that the ergogenic effects of the energy drink were due to the caffeine, with the other potential ingredients providing no additional benefits.
Mouth rinsing with caffeine may stimulate nerves with direct links to the brain, in addition to any caffeine absorption that occurs in the mouth. However, at this time, while caffeine mouth rinsing has been shown to improve short-duration, high-intensity, repeated sprinting and kicking efforts in normal and depleted muscle glycogen states (Kizzi et al., 2016; Pak et al., 2020), the majority of the literature has reported no ergogenic effect on aerobic exercise performance (Doering et al., 2014; Wickham & Spriet, 2018). In addition, caffeine mouth rinsing did not improve cognitive performance, although there was support for improvements in reaction time, cognitive control and countering mental fatigue (De Pauw et al., 2015; Van Cutsem et al., 2018). Caffeinated aerosol mouth and nasal sprays have also garnered recent interest as caffeine may stimulate nerves with direct brain connections and enter the blood via mucosal and pulmonary absorption. However, there is little support for an ergogenic effect as the delivery and/or effectiveness of caffeine delivered in this manner may be too small (De Pauw et al., 2017a, b).
CAFFEINE VARIABILITY AND GENETIC DISPOSITION
Studies that have published individual performance responses to caffeine ingestion have routinely reported large variability between subjects (Graham & Spriet, 1991; Myers & Cafarelli, 2005). In many cases, while group responses were statistically significant, some participants did not respond or responded very little to caffeine. Recently, there has been interest in trying to explain this variability by examining gene polymorphisms related to the metabolism of caffeine in the liver and the expression of adenosine receptors throughout the body. The liver enzyme, cytochrome P450, plays a large role in the metabolism of caffeine and a single nucleotide polymorphism at intron 1 of the cytochrome P450 (CYP1A2) gene influences the inducibility of this enzyme, such that people with the A variant metabolize caffeine faster and people with the C variant have slower rates of caffeine metabolism. Womack et al. (2012, 2015) examined whether the specific CYP1A2 polymorphism influenced the ergogenic effect of caffeine ingestion in trained cyclists. Their results, over a 40 km TT, suggested that individuals homozygous for the A allele (fast metabolizers) of this polymorphism had a larger ergogenic effect vs. placebo (71.6 + 4.3 vs. 75.1 + 6.1 min) than individuals with the C allele (71.6 + 4.4 vs. 73.1 + 4.5 min) following caffeine ingestion (Womack et al., 2012, 2015). Guest et al. (2018) extended this work and examined the ergogenic effects of three caffeine doses (2, 4 and 6 mg/kg bm) on 10 km cycling time trial performance in 101 competitive male athletes who were genotyped into AA, AC and CC CYP1A2 gene variant groups. In the AA genotype, performance time was improved by 6.8 % with 4 mg/kg bm and 4.8% with 2 mg/kg bm caffeine, while the CC genotype subjects performance time decreased by 13.7% with 4 mg/kg bm caffeine, and the AC group was unaffected by caffeine. However, many of the athletes in this study were not familiar with cycling as the performance test and the number of subjects in the CC group was very low (n=8). It should be noted, however, that not all studies have reported an effect of this gene polymorphism on performance, as reviewed by Southward et al. (2018). Additional work with a variety of athletes and sport-specific performance tests, and larger numbers in the CC groups is needed to conclusively determine the significance of the relationship between the metabolism and ergogenic effects of caffeine.
There has also been recent interest in whether polymorphisms of the ADORA2A gene, that encodes for the A2A subtypes of the adenosine receptor, may contribute to the variability in the ergogenic response to caffeine ingestion (Grgic et al., 2020b). One study has reported that the ergogenic effects of caffeine were only present in people with the TT genotype and not with the C allele (Loy et al., 2015). However, Grgic et al. (2020b) studied the response of 25 subjects, who were all ADORA2A C allele carriers (CC/CT genotype), to the ingestion of 3mg/kg bm caffeine using a large number of exercise performance tests (movement velocity, power output and muscle endurance during the bench press, jump height, Wingate test, etc.). Caffeine was ergogenic in this group in 21/25 measured variables and comparable to the benefits of previous reports where the population was not genotype specific, in direct contrast to the results of Loy et al. (2015). Clearly, more research is needed to determine if ADORA2A gene polymorphisms predict a portion of the variability in the ergogenic response to caffeine.
PRACTICAL APPLICATIONS
- When considering whether to use caffeine as a potential ergogenic aid, athletes should begin with low caffeine doses of ~100-200 mg (~1.5-3 mg/kg bm). Higher doses do not appear to confer additional advantages.
- As the response to caffeine consumption is highly variable, athletes need to trial the use of caffeine in training before moving to competitions.
- The ergogenic effects of caffeine are generally independent of habitual caffeine use, training status, dietary intake, gender, hydration status and exercise modality, but results in the heat are less clear (see Burke et al., 2013; Spriet, 2014).
- Caffeine can be administered in capsules, coffee, sports and energy drinks, gum, gels, bars and dissolvable mouth strips with ergogenic effects. Mouth rinsing with caffeine or aerosol caffeine administration is less likely to produce an ergogenic effect.
- It is not yet clear whether genetic polymorphisms can explain the inter-individual variability associated with the ergogenic effects of caffeine supplementation.
SUMMARY
Contemporary caffeine research has determined that lower doses of caffeine (<3 mg/kg bm, ~200 mg) are ergogenic in a wide variety of exercise and sport situations in recreationally and well-trained men and women. Higher doses are associated with altered physiological responses to exercise and additional side effects and do not confer additional benefits. The mechanisms that explain the ergogenic effects of caffeine appear to result from adenosine receptor antagonism in the CNS and PNS. While the administration of caffeine in capsules, tablets and coffee has been commonly used in research studies, caffeine can be delivered in many alternate forms with similar ergogenic effects. Recent work has examined whether polymorphisms in genes encoding for caffeine metabolizing enzymes and adenosine receptor subtypes can account for the inter-individual variability to caffeine administration, but additional research is needed to conclusively determine if genetic predisposition can predict the ergogenic effects of caffeine.
REFERENCES
Bowtell, J.L., M. Mohr, J. Fulford, S.R. Jackman, G. Ermidis, P. Krustrup, and K.N. Mileva (2018). Improved exercise tolerance with caffeine is associated with modulation of both peripheral and central neural processes in human participants. Front. Nutr. 5:6.
Burke, L.M. (2008). Caffeine and sports performance. Appl. Physiol. Nutr. Metab. 33:1319–1334.
Burke, L., B. Desbrow, and L. Spriet (2013). Caffeine for sports performance. Human Kinetics, Champaign, Illinois, USA.
Chia, J.S., L.A. Barrett, J.Y. Chow, and S.F. Burns (2017). Effects of caffeine supplementation on performance in ball games. Sports Med. 47:2453-2471.
Clarke, N.D., N.A. Kirwan, and D.L. Richardson (2019). Coffee ingestion improves 5 km cycling performance in men and women by a similar magnitude. Nutrients 25:11.
Costill, D.L, G. Dalasky, and W. Fink (1978). Effects of caffeine ingestion on metabolism and exercise performance. Med. Sci. Sports 10:155-158.
Cox, G.R., B. Desbrow, P.G. Montgomery, M.E. Anderson, C.R. Bruce, T.A. Macrides, D.T. Martin, A. Moquin, A. Roberts, J.A. Hawley, and L.M. Burke (2002). Effect of different protocols of caffeine intake on metabolism and endurance performance. J. Appl. Physiol. 93:990–999.
Cureton, K.J., G.L. Warren, M.L. Millard-Stafford, J.E. Wingo, J. Trilk, and M. Buyckx (2007). Caffeinated sports drink: ergogenic effects and possible mechanisms. Int. J. Sport Nutr. Exerc. Metab. 17:35–55.
Davis, J.M., Z. Zhao, and H.S. Stock (2003). Central nervous system effects of caffeine and adenosine on fatigue. Am. J. Physiol. 284:R399–R404.
De Pauw, K., B. Roelands, and K. Knaepen (2015). Effects of caffeine and maltodextrin mouth rinsing on P300, brain imaging, and cognitive performance. J. Appl. Physiol. 118:776–782.
De Pauw, K., B. Roelands, J. Van Cutsem, U. Marusic, T. Torbeyns, and R. Meeusen (2017a). Electro-physiological changes in the brain induced by caffeine or glucose nasal spray. Psychopharm. 234:53–62.
De Pauw, K., B. Roelands, J. Van Cutsem, L. Decroix, A Valente, K. Taehee, R.B. Lettan, A.E. Carrillo, and R. Meeusen (2017b). Do glucose and caffeine nasal sprays influence exercise or cognitive performance? Int. J. Sports Physiol. Perform. 12:1186-1191.
Desbrow, B., C. Biddulph, B. Devlin, G.D. Grant, S.D. Anoopkumar-Dukie, and M.D. Leveritt (2012). The effects of different doses of caffeine on endurance cycling time trial performance. J. Sports Sci. 30:115-120.
Doering, T.M., J.W. Fell, M.D. Leveritt, B. Desbrow, and C.M. Shing (2014). The effect of a caffeinated
mouth-rinse on endurance cycling time-trial performance. Int. J. Sport Nutr. Exerc. Metab. 24:90–97.
Fredholm, B.B. (1995). Adenosine, adenosine receptors and the actions of caffeine. Pharmacol. Toxicol. 76:93–101.
Gallo-Salazar, C., F. Areces, J. Abian-Vicen, B. Lara, C. Gonzalez-Millan, J. Portillo, V. Munoz, D. Juarez, and J. Del Coso (2015). Enhancing physical performance in elite junior tennis players with a caffeinated energy drink. Int. J. Sports Physiol. Perform. 10:305-310.
Graham, T.E., and L.L. Spriet (1991). Performance and metabolic responses to a high caffeine dose during prolonged exercise. J. Appl. Physiol. 71:2292–2298.
Graham, T.E., and L.L. Spriet (1995). Metabolic, catecholamine and exercise performance responses to varying doses of caffeine. J. Appl. Physiol. 78:767-774.
Grgic, J., F. Sabol, S. Venier, I. Mikulic, N. Bratkovoc, B.J. Schoenfeld, C. Pickering, D.J. Bishop, Z. Pedisic, and P. Mikulic (2020a). What dose of caffeine to use: Acute effects of 3 doses of caffeine on muscle endurance and strength. Int. J. Sports Physiol. Perform. 15:470-477.
Grgic, J., C. Pickering, D.J. Bishop, J. Del Coso, B.J. Schoenfeld, G.M. Tinsley, and Z. Pedisic (2020b). ADOR2A C allele carriers exhibit ergogenic responses to caffeine supplementation. Nutrients 11:12.
Guest, N., P. Corey, J. Vescovi, and A. El-Sohemi (2018). Caffeine, CYP1A2 genotype, and endurance performance in athletes. Med. Sci. Sport Exerc. 50:1570–1578.
Hodgson, A.B., R.K. Randell, and A.E. Jeukendrup (2013). The metabolic and performance effects of caffeine compared to coffee during endurance exercise. PLoS One 8:e59561.
Ivy, J.L., D.L. Costill, W.J. Fink, and R.W. Lower (1979). Influence of caffeine and carbohydrate feedings on endurance performance. Med. Sci. Sports 11:6-11.
Kalmar, J.M., and E. Cafarelli (2004). Caffeine: a valuable tool to study central fatigue in humans. Exerc. Sports Sci, Rev. 32:143–147.
Kamimori, G.H., C.S. Karyekar, R. Otterstetter, D.S. Cox, T.J. Balkin, G.L. Belenky, and E.D. Eddington. (2002). The rate of absorption and relative bioavailability of caffeine administered in chewing gum versus capsules to normal healthy volunteers. Int. J. Pharm. 234:159–167.
Kizzi J., A. Sum, F.E. Houston, and L.D. Hayes (2016). Influence of a caffeine mouth rinse on sprint cycling following glycogen depletion. Eur. J. Sport Sci. 16:1087–1094.
Lane, S.C., J.A. Hawley, B. Desbrow, A.M. Jones, J.R. Blackwell, M.L. Ross, A.J. Zemski, and L.M. Burke (2014). Single and combined effects of beetroot juice and caffeine supplementation on cycling time trial performance. Appl. Physiol. Nutr, Metab. 39:1050-1057.
Lara, B., D. Ruiz-Vicente, F. Areces, J. Abian-Vicen, J.J. Salinero, C. Gonzalez-Millan, C. Gallo-Salazar, and J. Del Coso (2015). Acute consumption of a caffeinated energy drink enhances aspects of performance in sprint swimmers. Br. J. Nutr. 114:908-914.
Loy, B.D. P.J. O’Connor, J.B. Lindheimer, and S.F. Covert (2015). Caffeine is ergogenic for adenosine A2A receptor gene (ADORA2A) T allele homozygotes: A pilot study. J. Caff. Res. 5:73–81.
Madden, R.F., K.A. Erdman, J. Shearer, L.L. Spriet, R. Ferber, A.T. Kolstad, J.L. Bigg, A.S.D. Gamble, and L.C. Benson (2019). Effects of low dose caffeine supplementation on measures of exertion, skill performance and physicality in ice hockey. Int. J. Sports Physiol. Perform. 14:1422-1429.
Meyers, B.M., and E. Cafarelli (2005) Caffeine increases time to fatigue by maintaining force and not by altering firing rates during submaximal isometric contractions. J. Appl. Physiol. 99:1056-1063.
Pak, I.E, M. Cug, S.L. Volpe, and C.M. Beaven (2020). The effect of carbohydrate and caffeine mouth rinse on kicking performance in competitive Taekwondo athletes during Ramadan. J. Sports Sci. 38:795-780.
Pasman, W.J., M.A. VanBaak, A.E. Jeukendrup, and A. DeHaan (1995). The effect of different dosages of caffeine on endurance performance time. Int. J. Sports Med. 16:225–230.
Paton, C.D., T. Lowe, and A. Irvine (2010). Caffeinated chewing gum increases repeated sprint performance and augments increases in testosterone in competitive cyclists. Eur. J. Appl. Physiol. 110:1243-1250.
Pitchford, N.W., J.W. Fell, M.D. Leveritt, B. Desbrow, and C.M. Shing (2014). Effect of caffeine on cycling time-trial performance in the heat. J. Sci. Med. Sport. 17:445-449.
Pickering, C., and J. Grgic (2019). Caffeine and exercise: What next? Sports Med. 49:1007-1030.
Rivers, W.H., and H.N. Webber (1907). The action of caffeine on the capacity for muscular work. J. Physiol. 36:33-47.
Quinlivan, A., C. Irwin, G.D. Grant, S. Anoopkumar-Dukie, T. Skinner, M. Leveritt, and B. Desbrow (2015). The effects of Red Bull energy drink compared with caffeine on cycling time-trial performance.
Int. J. Sports Physiol. Perform. 10:897-901.
Salinero, J.J., B. Lara, and J. Del Coso (2019). Effects of acute ingestion of caffeine on team sports performance: a systematic review and meta-analysis. Res. Sports Med. 27:238-256.
Skinner, T.L., B. Desbrow, J. Arapova, M.A. Schaumberg, J. Osborne, G.D. Grant, S. Anoopkumar-Dukie, and M.D. Leveritt (2019). Women experience the same ergogenic response to caffeine as men. Med. Sci. Sports Exerc. 51:1195-1202.
Southward, K., K. Rutherford-Markwick, C. Badenhorst, and A. Ali (2018). The role of genetics in moderating the inter-individual differences in the ergogenicity of caffeine. Nutr. 12:741.
Spriet, L.L. (2014). Exercise and sport performance with low doses of caffeine. Sports Med. 44:S175-S184.
Stevenson, E.J., P.R. Hayes, and S.J. Allison (2009). The effect of a carbohydrate-caffeine sports drink on simulated golf performance. Appl. Physiol. Nutr. Metab. 34:681–688.
Talanian, J.L., and L.L. Spriet (2016). Low and moderate doses of caffeine late in exercise improve performance in trained cyclists. Appl. Physiol. Nutr. Metab. 41:850-855.
Van Cutsem, J., K. De Pauw, S. Marcora, R. Meeusen, and B. Roelands (2018). A caffeine-maltodextrin mouth rinse counters mental fatigue. Psychopharm. 235:947-958.
Whalley, P.J., C.G. Dearing, and C.D. Paton (2019). The effects of different forms of caffeine supplement on 5-km running performance. Int. J. Sports Physiol. Perform. 15:390-394.
Wickham, K.A., and L.L. Spriet (2018). Administration of caffeine in alternate forms. Sports Med 48:S79-S91.
Womack, C.J., M.J. Saunders, M.K. Bechtel, D.J. Bolton, M. Martin, N.D. Luden, W. Dunham, and M. Hancock (2012). The influence of a CYP1A2 polymorphism on the ergogenic effects of caffeine. J. Int. Soc. Sports Nutr. 9:7.
Womack, C.J., M.J. Saunders, M.K. Bechtel, D.J. Bolton, M. Martin, N.D. Luden, W. Dunham, and M. Hancock (2015). Erratum to: The influence of a CYP1A2 polymorphism on the ergogenic effects of caffeine. J. Int. Soc. Sports Nutr. 12:24.
Figure Legends
Fig. 1. A: Effects of ingesting no caffeine (placebo) or 3, 6 or 9 mg/kg body mass of caffeine (dose) on running time to exhaustion at ~85% maximum oxygen uptake. Bars with different letters are significantly different and bars with the same letter are not significant. B: Plasma caffeine concentrations for 1 h at rest and during exercise to exhaustion at ~85% of maximum oxygen uptake in recreationally active subjects after the consumption of a placebo (open circles), or 3 (filled squares), 6 (filled triangles) or 9 (filled circles) mg/kg body mass of caffeine. Data are means ± standard error (n = 8). Exh, exhaustion (reproduced from Graham & Spriet 1995, with permission)
Fig. 2. The effects of ingesting caffeine on cycling time to complete a 5 kJ/kg body mass time trial (TT) following 120 min of submaximal cycling (caffeine or placebo ingestion at 80 min). Bars represent mean data for each treatment (means + SE, n = 15). Lines represent individual TT performances across each treatment. CAF1, 100 mg caffeine; CAF2, 200 mg caffeine; PL, placebo; *, significantly faster than PL; †, significantly faster than CAF1. (reproduced from Talanian & Spriet, 2016, with permission)