KEY POINTS
- Women have lower whole body sweating rate (WBSR) and sweat sodium concentrations as a population than men, but in most environments these differences are attributed to lower absolute workloads. During the luteal phase, there is an increase in the body core temperature threshold for the onset of sweating, but there are no differences in WBSR across menstrual cycle phases.
- Significant hypohydration before and during exercise is less common in women than men. Exercise-associated hyponatremia is more common in women but is attributed more so to drinking behavior, smaller body size, and longer race times, than sex per se.
- During the luteal phase, estrogen decreases the osmotic threshold for thirst and vasopressin release and progesterone may increase aldosterone production. However, the menstrual cycle phase seems to have no impact on ad libitum rehydration, overall fluid balance, or fluid retention at rest and during exercise.
- Women exhibit similar physiological responses to dehydration during exercise-heat stress compared with men when matched for fitness, heat acclimation, and body fat percentage.
- Hypohydration (≥ 2% body mass loss), can adversely affect team sport skill, cognitive, and endurance performance in women as it does in men, albeit fewer studies have been conducted with female athletes.
INTRODUCTION
A significant amount of research has been conducted to understand body water regulation and the effects of hypohydration on thermoregulation, cardiovascular function, and performance during exercise in heat. However, women have been underrepresented in hydration research, accounting for less than ~30% of subjects tested in recent studies (Nuccio et al., 2017). Based on the existing body of literature, it is recommended that fluid replacement strategies should be personalized per individual sweating rates and exercise conditions, with the goal of preventing ≥ 2% body mass loss (BML) to maintain performance (McDermott et al., 2017). However, it is unclear whether additional
considerations should be made for the female sex when developing a personalized fluid replacement plan. The purpose of this Sports Science Exchange (SSE) article is to provide a narrative review of hydration studies conducted on women and suggest where future research may be needed. Potential effects of the menstrual cycle phase as well as differences between men and women will be discussed, while also distinguishing between population differences and true sex differences. This review will include fluid loss (sweating rate and sweat composition), rehydration (fluid intake, absorption, and retention), and the physiological/performance effects of hypohydration during exercise. The primary focus here is adult female subjects (women) with normal menstrual cycles. There is limited research on hydration with respect to hormonal contraceptive use or menstrual cycle irregularities.
FEMALE PHYSIOLOGY AND PHYSICAL CHARACTERISTICS
On average women have a smaller body size and a higher body fat percentage than men. Because adipose tissue has a lower percentage of water (~10%) than fat-free mass (~74%), body water makes up a lower overall percentage of a women’s mass (Visser et al., 1997). Thus, water loss via sweating represents a slightly larger percentage of body water loss in women than men (Table 1).
As a population, women also have lower aerobic capacities and poorer heat acclimation status, both of which influence thermoregulatory sweat loss and other physiological responses to exercise-heat stress (Kenney, 1985). Therefore, it is important to consider fitness and heat acclimation when interpreting results of studies comparing responses of men and women to exercise heat stress and hypohydration.
Fluctuations in female reproductive hormone concentrations occur throughout the menstrual cycle and influence certain aspects of body temperature regulation. For instance, women have an increased body core temperature at rest and during exercise in the midluteal phase compared with the early follicular phase (Stephenson & Kolka, 1985). The increased body core temperature is likely mediated by higher progesterone concentrations in the luteal phase, which increases the set point for heat dissipation mechanisms. This means that cutaneous vasodilator and sweating responses are initiated ~0.5°C higher compared with the early follicular phase (Kolka & Stephenson, 1997). There are also some hormone mediated effects on the regulation of body water and electrolytes. During the luteal phase, estrogen can decrease the osmotic threshold for thirst and vasopressin release (Stachenfeld et al., 1999) and progesterone can increase aldosterone production (Quinkler et al., 2002). While there are clear effects of female sex hormones on body temperature and fluid regulation, they do not necessarily translate into significant differences in overall sweat loss or fluid/electrolyte balance between men and women, or across the menstrual cycle. The remaining sections of this SSE will review the available studies investigating sweat losses, sweat composition, rehydration, and the physiological/performance effects of hypohydration in women. The findings are summarized in Figure 1.
FLUID LOSS
Eccrine Gland Density and Regional Distribution of Sweat
During exercise, heat is produced by the contracting muscles as a byproduct of metabolism. Evaporation of sweat is the primary avenue of heat loss to maintain thermal balance during exercise. Whole body sweating rate (WBSR) is a product of the density of active eccrine sweat glands and the secretion rate per gland in response to thermal stimuli. Sweat gland density is generally higher in women than men (due in part to lower body surface area). However, the distribution of sweat glands in women has a similar pattern to that found in men, with the back having the highest density and the chest the lowest (Bar-Or et al., 1968). With respect to regional sweating rates, men and women have similar ‘‘high’’ and ‘‘low’’ sweat regions with the highest rates on the back and the lowest rates on the extremities (Baker et al., 2018). However, there are slight differences between sexes in the overall pattern of sweat distribution. For example, one study found that men had a higher distribution of sweat toward the torso, while in women, the arms, hands, and feet contributed relatively more to total sweat loss (Smith & Havenith, 2012). Interestingly, women may use a higher percentage of their sweat glands while excreting less sweat per gland. This greater sweating efficiency may reduce the amount of “wasted sweat”– i.e., sweat that drips off the skin and therefore does not contribute to evaporative cooling of the body. From a hydration perspective, less wasted sweat may be an advantage for women in hot-humid environments, where sweat drippage is more likely to occur because of a reduced vapor pressure gradient for evaporation. By having a lower sweating rate in hot-humid conditions women lose less fluid and limit hypohydration, while men may drip more sweat from their bodies and become more dehydrated (Avellini et al., 1980; Shapiro et al., 1980).
Sweating Rate
It is often reported that men exhibit higher sweating rates than women. However, research investigating the effect of sex on sweat loss during exercise has often been confounded by the exercise intensity prescribed to compare groups. Higher WBSR observed in men (e.g., in cross-sectional studies) can usually be attributed to higher body mass and absolute exercise intensities (Sawka et al., 2007; Smith & Havenith, 2012). These factors increase metabolic heat production, which in turn increases the evaporative requirement for heat balance (amount of evaporated sweat needed to regulate body core temperature) (Gagnon et al., 2013). Recent studies have isolated the effect of sex from differences in metabolic heat production in adults, by matching subjects for body mass, surface area, and metabolic heat production. This research suggests that whole-body sweat production is similar between men and women in most conditions – i.e., at fixed rates of heat production up to 250 W/m2. However, at higher heat loads, women have a lower sweating capacity than men. These sex differences are evident only above a certain combination of environmental conditions (35 – 40°C, 12% relative humidity) and metabolic heat production (300 – 500 W/ m2) (Gagnon & Kenny, 2012; Jay, 2014). The sex difference in maximal sweating capacity is due to properties of the sweat gland related to cholinergic responsiveness, which leads to a lower sweat output per gland. Nonetheless, real-world evidence of a thermoregulatory disadvantage leading to heat intolerance in women during exercise is not apparent. The reader is referred to other papers for more information on sex comparisons with respect to thermoregulation and heat tolerance (Gifford et al., 2019; Yanovich et al., 2020). Taken together, women are not at a thermoregulatory disadvantage compared with men for most activities and environmental conditions typically encountered. With respect to WBSR, it is important to highlight the significant interindividual variation as well as potential sex differences. Figure 2 shows frequency histograms for WBSR in male (1565 sweat tests) and female (379 sweat tests) participants measured across a wide range of sports/activities and environmental conditions in the laboratory or field by the Gatorade Sports Science Institute (GSSI) (Baker et al., 2022a). On average, WBSR was lower in female (0.8 L/h) versus male (1.2 L/h) subjects, but the mean difference between sexes (0.4 L/h) was much less than the overall range (> 2 L/h) in WBSR. Thus, it stands to reason that, regardless of sex, assessments of WBSR should be conducted to learn about his/her individual fluid replacement needs. Several studies have investigated the sweating response to exerciseheat stress across the menstrual cycle. Overall, this research suggests that, although the menstrual cycle may modulate the control of sweating there are no effects on WBSR. During the luteal phase, there is an upward shift in the body core temperature threshold for initiation of regional sweating during exercise-heat stress, particularly in untrained women (Kolka & Stephenson, 1989; Kuwahara et al., 2005). However, several studies have reported no effects of female sex hormones on WBSR (Stachenfeld & Taylor, 2009) and no differences in WBSR across the menstrual cycle phases (Freemas et al., 2023; Giersch et al., 2020; Notley et al., 2019). This finding is consistent across a range of exercise intensities and environmental conditions.
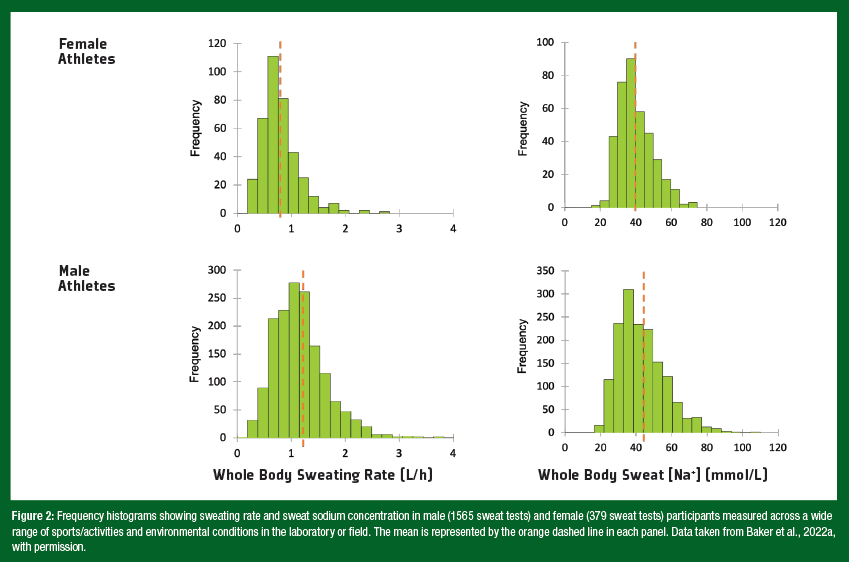
Sweat Electrolyte Concentrations
As a population, sweat sodium and chloride concentrations ([Na+] and [Cl–]) tend to be slightly lower in women than men (Baker et al., 2018; Meyer et al., 1992), due in part to the confounding effect of absolute exercise intensity and differences in sweating rate between sexes (as discussed above). Sweating rate is an important factor determining sweat [Na+] and [Cl–]. This is because the rate of Na+ and Cl– reabsorption in the sweat duct is flow dependent. As sweating rate increases the rate of Na+ and Cl– secretion in precursor sweat increases proportionally more than the rate of Na+ and Cl– reabsorption along the duct, and therefore leads to higher final sweat [Na+] and [Cl–]. Thus, there is a direct relation between sweating rate and sweat [Na+] and [Cl–] (Buono et al., 2008). Given the well-established relation between sweat flow rate and sweat electrolyte concentrations, it follows that any factors stimulating acute increases in sweating rate, such as increased exercise intensity, would result in higher sweat [Na+] and [Cl–]. Accordingly, when studies account for exercise intensity and/or sweating rate, there is no effect of sex on sweat [Na+] and [Cl–] (Baker et al., 2022a). There are also no effects of female sex hormones on sweat [Na+] (Stachenfeld & Taylor, 2009). Like WBSR, it is also important to note the significant interindividual variation in sweat [Na+]. As illustrated in Figure 2, average sweat [Na+] measured across a wide range of sports/activities and environmental conditions was lower in female (40 mmol/L) versus male (43 mmol/L) subjects. However, the mean difference between sexes (3 mmol/L) was much less than the overall range (> 80 mmol/L) in sweat [Na+] (Baker et al., 2022a). Due to the variation in sweat [Na+] as well as WBSR, individual sweat testing should be conducted to estimate sweat Na losses for each environment (e.g., indoor/outdoor, summer/autumn season) and exercise condition (light/high-intensity practice) to guide fluid replacement plans. While exercise intensity and environment are the main determinants of WBSR and sweat [Na+], other factors such as heat acclimation and aerobic training also play important roles in intraand interindividual variation for both men and women.
REHYDRATION PROCESS
Fluid Intake and Fluid Balance
Regulation of Thirst Sensation. Exercise-induced hypohydration results in hyperosmotic (increased plasma osmolality) hypovolemia (decreased plasma volume) because sweat is hypotonic to the blood (Kozlowski & Saltin, 1964). Both plasma osmolality and volume are tightly regulated by reflex adjustments mediated by nerves and hormones to help restore body water. For example, in response to hyperosmolality, physiological thirst is stimulated to increase fluid intake. The onset of thirst occurs once plasma osmolality reaches a certain threshold or set point. Although there is significant interindividual variability, the average osmotic threshold for thirst is similar between men and women during the follicular phase. During the luteal phase, estrogen mediates a downward shift in the osmotic threshold (by ~5 mosm/kg) for thirst in women (Stachenfeld, 2014; Vokes et al., 1988). One might hypothesize that the set point shift could lead to more fluid intake during the luteal versus follicular phase. However, a recent study found no differences in ad libitum fluid intake during exercise between the early follicular, late follicular, and mid-luteal phases of the menstrual cycle (Freemas et al. 2023). Furthermore, as discussed in the next sections, there seem to be minimal effects of the menstrual cycle or sex on overall fluid balance. Where differences are noted, evidence points to physical and behavioral explanations rather than hormone-mediated changes in physiological thirst. Pre-Exercise Fluid Intake Behavior and Hydration Status. Several studies have attempted to estimate hydration status and gauge adequacy of fluid intake behavior leading up to a practice session. To do this, investigators typically measured urine specific gravity (USG) of samples collected from athletes before exercise. Although spot USG assessment has limitations as a marker of hydration status, it does give an indication of whether renal water conservation mechanisms have been activated (as suggested by a high USG, e.g., ≥ 1.020) (see Baker et al., 2022a for review). Overall, studies suggest that USG values ≥ 1.020 may be less prevalent in female athletes. For example, at an NCAA Division I University, 47% of the male athletes (n=138) had prepractice USG ≥ 1.020, whereas only 28% of female athletes’ (n=125) urine samples were ≥ 1.020 (Volpe et al., 2009). In a systematic review of 24 studies that measured pre exercise USG in soccer players, elevated USG was found in 66% of male players (n=468) and only 47% of female players (n=79) (Chapelle et al., 2020). However, in another study, 79% of NCAA Division II basketball players’ samples had USG > 1.020 before offseason conditioning and preseason practices, with no significant differences between men (n=11) and women (n=11) (Thigpen et al., 2014).
Exercise Fluid Intake Behavior and Hydration Status.
When there is a mismatch between sweat loss and fluid intake this imbalance leads to a body water deficit (hypohydration) or surplus (hyperhydration). Acute changes in fluid balance (i.e., hydration status) during/after exercise are assessed by comparing the individual’s body mass to baseline or pre-exercise values. For example, 2% hypohydration is defined as a water deficit equal to 2% of body mass. This method has limitations since a small portion of body mass loss during activity is from non-water sources (e.g., substrate oxidation), but is the simplest and most used index in real-time.
Several observational field studies have measured ad libitum fluid intake and fluid balance during exercise in women. In team or skill sports, the level of hypohydration accrued throughout exercise is usually mild, with mean fluid balance reported to be less than 1 – 2% BML (Nuccio et al., 2017). However, where the full range in body mass change has been reported significant hypohydration (up to 2 – 2.5%) has been observed in some elite female cricket, field hockey, and basketball athletes during competition (Brandenburg & Gaetz, 2012; MacLeod & Sunderland, 2009; Soo & Naughton, 2007).
In endurance sports, such as marathons and race walking, body mass loss tends to be greater in men compared with women (Periard et al., 2017; Rehrer et al., 1989). However, significant hypohydration (> 2% BML) has also been reported in female endurance athletes (Rehrer et al., 1989). On the other hand, the incidence of exercise-associated hyponatremia (EAH; blood sodium < 135 mmol/L) is higher in women than men (Almond et al., 2005). The primary cause of EAH is water overload (i.e., overdrinking relative to sweat losses during exercise) and studies have found that women tend to overdrink more so than men (Baker et al., 2005; Hew, 2005). Other factors that may increase a woman’s risk for EAH include lower body mass and total body water (Stachenfeld & Taylor, 2009), body mass index extremes, and longer marathon race times (Almond et al., 2005). When these factors are accounted for in the statistical analyses, the sex difference in EAH incidence is nullified (Almond et al., 2005).
Taken together, there is considerable variation in fluid intake behavior, with some women experiencing hypohydration and others overestimating their fluid needs during exercise. Thus, care should be taken to determine individual fluid replacement needs for a given endurance event. This will help inform an appropriate drinking strategy so that significant overdrinking and under drinking can be avoided.
Fluid Delivery
After fluid ingestion, the next critical step in the rehydration process is fluid delivery – i.e., gastric emptying and intestinal absorption of fluid into circulation. It is unclear whether the gastric emptying rate of ingested fluids is influenced by sex, as the few studies available have reported mixed results in healthy subjects at rest (Caballero-Plasencia et al., 1999; Hellmig et al., 2006). It has been consistently reported, however, that gastric emptying of fluids at rest is not affected by the menstrual cycle phase (Gill et al., 1987; Horowitz et al., 1985). No studies have investigated the effects of sex or menstrual cycle phase on gastric emptying of fluids during exercise. In addition, no studies, to the authors’ knowledge, have assessed sex or menstrual cycle phase effects on intestinal fluid absorption at rest or during exercise. While the effect of sex on fluid delivery has not been well-studied, it is clear that the composition of fluid (the amount and type of carbohydrate) ingested plays a significant role in the rate of gastric emptying and intestinal absorption (Leiper, 2015). For example, hypertonic beverages with relatively high energy density (≥ 8% carbohydrate) reduce gastric emptying rate and appearance of fluid in the blood. In turn, the slower fluid delivery is associated with an increased risk for gastrointestinal discomfort during intermittent, high-intensity exercise. It is important to note that the effect of higher concentrated beverages on fluid delivery has been demonstrated in both men and women (Evans et al., 2011; Shi et al., 2004).
Fluid Retention
Once fluid is absorbed into the circulation, the next important step in the rehydration process is retention of the ingested fluid in the body, particularly the vascular space, to completely restore water balance. Arginine vasopressin (AVP) and aldosterone play important roles in fluid retention by increasing the renal reabsorption of water and sodium, respectively. During the luteal phase, estrogen mediates a downward shift in the osmotic threshold for AVP release (Stachenfeld et al., 1999) and progesterone is thought to mediate an increase in aldosterone concentration (Quinkler et al., 2002). These changes might be expected to result in greater fluid and sodium retention in women during the luteal phase. However, several studies have found no effects of sex (Sollanek et al., 2018), sex hormones (Calzone et al., 2001; Rodriguez-Giustiniani & Galloway, 2019; Stachenfeld et al., 1999), or menstrual cycle phase (Freemas et al., 2023; Maughan et al., 1996) on plasma volume or whole-body fluid retention. These results suggest that female sex hormones alter the osmotic operating point for body water balance, but do not lead to greater fluid retention. Taken together, the available literature suggests that replacement of exercise induced sweat losses is not affected by the normal menstrual cycle in eumenorrheic women. However, as many active women can have amenorrhea or oligomenorrhea due to disturbances in hormonal status, the responses of those individuals may be different and requires future research (Rodriguez-Giustiniani et al., 2022).
PHYSIOLOGICAL RESPONSES TO HYPOHYDRATION
Hypohydration is associated with impaired circulatory and thermoregulatory function during exercise, particularly when accompanied by heat stress (Sawka et al., 2007). Hypovolemia leads to a decrease in stroke volume and a compensatory increase in heart rate to maintain a given cardiac output during exercise. Increased plasma osmolality decreases the capacity to sweat and attenuates skin blood flow. In turn, impairment of heat dissipating mechanisms results in a larger increase in body core temperature for a given work rate compared with euhydration. Hypohydration is also associated with a decline in muscle blood flow and altered energy metabolism, including increased lactate, muscle glycogenolysis, and carbohydrate oxidation during prolonged exercise (Gonzalez-Alonso et al., 1998; Sawka et al., 2007). Although most studies have been conducted in men, similar physiological responses to hypohydration have also been observed in women (Logan-Sprenger et al., 2012; Watanabe et al., 2020; Wickham
et al., 2021). Few studies, however, have made direct comparisons to investigate potential sex differences in the physiological response to hypohydration. Sawka and colleagues investigated the effects of 5% BML in men and women during exercise in temperate, hot/dry, and hot/humid conditions. The investigators found no sex differences in the changes in plasma volume, heart rate, rectal temperature, or skin temperature in these studies (Cadarette et al., 1984; Sawka et al., 1983). A key consideration is that participants were acclimated to the heat prior to testing. In addition, men and women were matched for body fat percentage and VO2max, thereby removing any effect of these confounding factors. The balance of research also suggests there are no differences in plasma volume shifts between menstrual cycle phases during exercise (Rodriguez-Giustiniani et al., 2022).
One study compared the effect of mild hypohydration on body core temperature responses to exercise-heat stress in men and women (Giersch et al., 2021). In this study, subjects were asked to restrict fluid for 24 h prior to interval exercise (two 30-min blocks of exercise separated by 15 min of rest). There were no effects of sex or menstrual cycle phase on the level of hypohydration reached (~1% BML). However, when hypohydrated women experienced a faster increase in rectal temperature in the first block of exercise than men (0.06 °C/min vs. 0.03 °C/min), with no differences between the late follicular and midluteal phase. These results seem to suggest that women may be more sensitive to the thermoregulatory effects of hypohydration during the early stages of exercise in the heat (Giersch et al., 2021). It is important to note, however, that in this study men and women were not matched for aerobic fitness and women exercised at a higher percentage of their VO2max. These factors may account in part for the sex differences in rectal temperature responses observed in this study. Taken together, the limited number of studies available suggest minimal effects of sex in the physiological response to exercising in a hypohydrated state.
EXERCISE PERFORMANCE
Female athletes are under-represented in studies investigating hypohydration effects on exercise performance. According to a narrative review, only 30% of studies on hydration and team sports performance included female athletes (Nuccio et al., 2017). In cycling meta-analyses, women represented 10-18% of all subjects (Goulet, 2011; Holland et al., 2017). Further, women accounted for only 6% of the participants in studies included in a hypohydration meta-analysis on muscle endurance, strength, anaerobic power and capacity and vertical jumping ability (Savoie et al., 2015).
Based on the studies that have included women, it seems that hypohydration impairs performance as it does in men. That is, ≥ 2% BML is associated with impaired endurance and team sport performance as well as some aspects of strength and anaerobic power. For instance, 12-km trail race times in the heat were slower when runners (9 men, 8 women) were hypohydrated (4.3% BML) compared with when they were allowed to drink ad libitum (2.1% BML). The authors reported that there were no differences between the sexes in terms of how hypohydration impacted performance results (Casa et al., 2010). In another study that included both men (n=6) and women (n=2), 3% BML impaired endurance performance during a 30-min cycling time trial, in temperate but not cold conditions (Cheuvront et al., 2005). Research with female athletes has found mixed results with respect to hypohydration effects on team sports performance, but on balance suggests that ≥ 2% BML may impair skill, agility, and decision-making. Studies with female basketball players found that 2.1% and 2.3% BML were associated with lower field goal percentage (Brandenburg & Gaetz, 2012) and impaired lower body reactive agility (Hoffman et al., 2012), respectively, compared with euhydration. Two percent BML increased decision-making time in field hockey players (MacLeod & Sunderland, 2012), whereas 2.2% and 2.7% BML had no effect on skill performance in soccer (Ali et al., 2011) or tennis (Burke & Ekblom, 1984), respectively.
A few studies have tested the effect of hypohydration on muscular strength, endurance, and power in women. For example, in resistance-trained women, 3% BML had a negative impact on bench press 1 RM performance and perceptual feelings of recovery, while other measures including leg press 1 RM and vertical jump height were not impacted by hypohydration (Gann et al., 2021). In a study with physically active men (n=5) and women (n=5), 4% BML reduced muscle endurance during single-leg knee extension exercise (Montain et al., 1998). In another study, mild hypohydration induced by sauna exposure reduced explosive power during a squat jump in female (1.4% BML), but not male athletes (1.8% BML). Interestingly, the decrease in squat jump performance was linearly related to percentage BML among the six women tested (Gutierrez et al., 2003). Finally, in female ice hockey players 1.7% BML led to lower sprint power output during an intermittent high-intensity cycling protocol (Driscoll et al., 2020).
GENERAL HEALTH
The United States Institute of Medicine’s (IOM) Adequate Intake for total water, based on the median total water intake from the U.S. National Health and Nutrition Examination Survey data, is 2.7 L/d for women. The European Food Safety Authority (EFSA) defines Adequate Intake for total water as 2.0 L/d for women. Emerging hypotheses suggest that suboptimal habitual fluid intake may be associated with a higher risk for certain acute conditions and chronic diseases (Kavouras, 2019; Perrier, 2017). The reader is referred to SSE #223 on Hydration for Health and Wellness (Baker et al., 2022b) for a more thorough review of this topic. A health condition particularly relevant to female athletes is urinary tract infections (UTIs). Approximately half of adult women will have at least one UTI in their lifetime, 27% of whom will have a confirmed recurrence within 6 months. Some descriptive studies have found that lower daily fluid intake (≤ 1.0 – 1.4 L/d) was associated with an increased risk for UTI (Nygaard & Linder, 1997; Vyas et al., 2015). Increasing fluid intake increases urine flow, which is thought to help prevent the development of UTI by flushing the urinary tract of bacteria. In a randomized controlled trial with 140 premenopausal women suffering from recurrent cystitis, there was a 48% reduction in UTI episodes after increasing total fluid intake from 1.1 to 2.8 L/d for 12 months. An important secondary benefit was a reduction in antibiotics used to treat UTIs in the women who increased fluid intake (Hooton et al., 2018).
Cognition and Mood
Several studies suggest that reduced fluid intake leading to mild hypohydration can have negative effects on mood and certain aspects of cognition in women (Armstrong et al., 2012; D'Anci K et al., 2009; Pross et al., 2013; Stachenfeld et al., 2018). The detrimental effects of inadequate fluid intake seem to be applicable to free-living conditions, at rest, and during exercise. This is important because it suggests that appropriate hydration has implications for physically active women in their everyday lives as well as exercise performance. Moreover, it reiterates the importance of starting training/competition in a euhydrated state to avoid potential adverse cognitive or mood effects of hypohydration on the athlete’s mental readiness to perform.
A well-designed study with particular relevance to everyday hydration was published by Stachenfeld et al. (2018). This group measured the effects of fluid restriction (0.7 L/d) compared with fluid intake sufficient to meet the IOM and EFSA recommendations (2.6 L/d) on cognitive performance in free-living, female college students. Mild hypohydration (1.0% BML) accrued over 24 h of fluid restriction led to deficits in visual and working memory as well as executive function, but no change in mood or performance of simple tasks of reaction time or identification (Stachenfeld et al., 2018). In another study, 24 h fluid deprivation in young healthy women led to decreased alertness and increased sleepiness, fatigue, and confusion (Pross et al., 2013). Importantly, in these studies rehydration reversed the hypohydration-associated impairments in memory, executive function (Stachenfeld et al., 2018), alertness, and confusion (Pross et al., 2013), albeit not other measures of mood. One observational study analyzed the relation between habitual water intake (categorized as low: 1.5 L/d, moderate 2.25 L/d, and high: 3.1 L/d) and mood in 120 healthy young women over a 5-day period. Total mood disturbance was higher in women who habitually consumed low versus high daily total water (Munoz et al., 2015). It is important to note that this study did not measure hydration status; nonetheless, the results suggest that drinking less than the Adequate Intake for water may be associated with adverse mood states.
Fewer studies have investigated cognition and mood effects of hydration status in women during exercise. Mild hypohydration (1.4% BML) resulted in degraded mood, increased perception of task difficulty, lower concentration, and headache symptoms at rest and during exercise in a study with physically active women. However, most aspects of cognitive performance were not affected by hypohydration (Armstrong et al., 2012). In a study with college athletes, 1.8% BML decreased vigor, increased fatigue, and impaired vigilance compared with euhydration. There was a significant sex-by-hydration condition interaction effect on choice reaction time as women made more errors when hypohydrated, whereas men made fewer errors in the hypohydrated condition compared with the euhydrated condition (D'Anci K et al., 2009).
Interestingly, other studies have suggested potential sex differences in mood and cognitive responses to hydration status (Bethancourt et al., 2020; Suh et al., 2021; Szinnai et al., 2005). For example, in serial addition and Stroop (working memory, executive function) tasks, females had a slower reaction time whereas men had a faster reaction time after 24-h water deprivation resulting in 2.6% BML (Szinnai et al., 2005). In another study, total mood disturbance scores increased in women, but not men in response to cellular dehydration induced by hypertonic saline infusion (Suh et al., 2021). The explanation for these results is unclear but could be related to physiology and/or sociology differences between men and women. A potential physiological mechanism was suggested by Suh et al. (2021). In their study sex differences in total mood disturbance were related to elevated thirst and copeptin concentrations (surrogate for vasopressin) in women. Interestingly, in sociology research, it has been proposed that women are more willing to accept their moods and to self-report negative feelings than men (Bird & Rieker, 2008). Men might downplay the impact of fatigue, whereas women more readily communicate their symptoms (Courtenay, 2011).
FUTURE RESEARCH DIRECTIONS
The available research suggests that, like men, a women’s hydration plan should be designed to avoid ≥ 2% BML. However, the effect of lower levels of hypohydration (1 -2% BML) on women’s exercise performance is largely unknown. Graded hypohydration studies with men have generally found that performance is not negatively impacted by hypohydration until BML reaches ~2% (Baker et al., 2007; Cheuvront & Kenefick, 2014). Beyond 2% BML there is a progressive deterioration in performance as hypohydration worsens (Baker et al., 2007), which is accompanied by a progressive increase in thermoregulatory and cardiovascular strain (Montain & Coyle, 1992; Sawka et al., 1985). As discussed previously, a given percentage BML from sweat loss in women represents a slightly larger percentage of their body water compared with men. Therefore, it would be important to investigate the effect of mild progressive hypohydration (e.g., 1%, 1.5%, 2%, etc.) on performance in women. To determine if hydration recommendations should differ between sexes, direct (within study) comparisons are needed. Future work should also investigate potential sex differences in mood disturbance and cognitive impairment related to hypohydration. In addition, the effects of amenorrhea or oligomenorrhea, which are issues with many female athletes, on fluid balance should be investigated. Finally, although some of the studies discussed above included women on oral contraceptives, few studies have investigated the effects of hormonal contraceptives on hydration. Therefore, research on sweat loss and fluid balance in women taking different kinds of hormonal contraceptives is needed.
CONCLUSION
Although female sex hormones alter certain aspects of thermoregulation and body fluid regulation, there seem to be minimal effects of the menstrual cycle phase or sex per se on WBSR, fluid balance, or the physiological/performance effects of hypohydration. Instead, factors such as body size, absolute workload, aerobic fitness, heat acclimatization status, and behavioral differences generally play a larger role in determining sweat loss and fluid balance. Thus, hydration strategies should be tailored to the individual based on the athlete’s sweat losses and exercise/environmental conditions, with the overall goal of preventing significant fluid/electrolyte imbalances.
PRACTICAL APPLICATIONS
- Female athletes should drink enough fluid during exercise to avoid significant hypohydration, as ≥ 2% BML is associated with performance decrements in women.
- It is also important to advise female athletes to avoid body mass gain during/after exercise since women tend to be more likely to overdrink relative to sweat losses.
- There is considerable variation in sweating rates and sodium losses among female athletes. Individualized sweat testing should be conducted to guide the athlete’s hydration strategy.
- Significant hypohydration (≥ 2% BML) during exercise is not commonly observed in women. However, because women tend to have a higher body fat percentage, water lost in sweat may represent a slightly larger percentage of total body water and plasma volume in women than men. Therefore, more research is needed to determine the effect of milder levels of hypohydration (1 – 2% BML) on performance in female athletes.
- For women, the Adequate Intake (AI) for total water is approximately 2.0 L/d (EFSA) to 2.7 L/d (USDA). The AI does not include fluid intake needed to replace individual sweat losses, which may vary day-to-day depending upon exercise duration/intensity and the environment.
- Appropriate hydration in women can have everyday health benefits, including cognitive performance and mood. In addition, increasing habitual water intake can decrease the risk of recurrent UTIs
The author is employed by the Gatorade Sports Science Institute, a division of PepsiCo R&D.
The views expressed are those of the author and do not necessarily reflect the position or policy of PepsiCo, Inc.
REFERENCES
Ali, A., R. Gardiner, A. Foskett, and N. Gant (2011). Fluid balance, thermoregulation and
sprint and passing skill performance in female soccer players. Scand. J. Med. Sci.
Sports 21:437-445.
Almond, C.S., A.Y. Shin, E.B. Fortescue, R.C. Mannix, D. Wypij, B.A. Binstadt, C.N. Duncan,
D.P. Olson, A.E. Salerno, J.W. Newburger, and D.S. Greenes (2005). Hyponatremia
among runners in the Boston Marathon. N. Engl. J. Med. 352:1550-1556.
Armstrong, L.E., M.S. Ganio, D.J. Casa, E.C. Lee, B.P. McDermott, J.F. Klau, L. Jimenez, L.
Le Bellego, E. Chevillotte, and H.R. Lieberman (2012). Mild dehydration affects mood
in healthy young women. J. Nutr. 142:382-388.
Avellini, B.A., E. Kamon, and J.T. Krajewski (1980). Physiological responses of physically fit
men and women to acclimation to humid heat. J. Appl. Physiol. 49:254-261.
Baker, L.B., T.A. Munce, and W.L. Kenney (2005). Sex differences in voluntary fluid intake by
older adults during exercise. Med. Sci. Sports Exerc. 37:789-796.
Baker, L.B., K.A. Dougherty, M. Chow, and W.L. Kenney (2007). Progressive dehydration
causes a progressive decline in basketball skill performance. Med. Sci. Sports Exerc.
39:1114-1123.
Baker, L.B., C.T. Ungaro, B.C. Sopena, R.P. Nuccio, A.J. Reimel, J.M. Carter, J.R. Stofan, and
K.A. Barnes. (2018). Body map of regional versus whole body sweating rate and sweat
electrolyte concentrations in men and women during moderate exercise-heat stress. J.
Appl. Physiol. 24(5):1304-1318.
Baker, L.B., P.J.D. De Chavez, R.P. Nuccio, S.D. Brown, M.A. King, B.C. Sopena, and K.A.
Barnes (2022a). Explaining variation in sweat sodium concentration: effect of individual
characteristics and exercise, environmental, and dietary factors. J. Appl. Physiol.
133:1250-1259.
Baker, L.B., C.D. Rehm, and M.A. King (2022b). Hydration for health and wellness, Sports
Science Exchange. #223.
Bar-Or, O., L.I. Magnusson, and E.R. Buskirk (1968). Distribution of heat-activated sweat
glands in obese and lean men and women. Hum. Biol. 40:235-248.
Bethancourt, H.J., W.L. Kenney, D.M. Almeida, and A.Y. Rosinger (2020). Cognitive
performance in relation to hydration status and water intake among older adults,
NHANES 2011-2014. Eur. J. Nutr. 59:3133-3148.
Bird, C.E., and P.P. Rieker (2008). Gender and health: The effects of constrained choices and
social policies. 1st Edition. Cambridge University Press. 274 pages.
Sports Science Exchange (2023) Vol. 36, No. 237, 1- 10
8
Brandenburg, J.P., and M. Gaetz (2012). Fluid balance of elite female basketball players
before and during game play. Int. J. Sport Nutr. Exerc. Metab. 22:347-352.
Buono, M.J., R. Claros, T. Deboer, and J. Wong (2008). Na+ secretion rate increases
proportionally more than the Na+ reabsorption rate with increases in sweat rate. J.
Appl. Physiol. 105:1044-1048.
Burke, E.R., and B. Ekblom (1984). Influence of fluid ingestion and dehydration on precision
and endurance performance in tennis. Proceedings of the World Congress of Sports
Medicine, Vienna, 1982. Curr. Top. Sports Med. p. 379-388.
Caballero-Plasencia, A.M., M. Valenzuela-Barranco, J.L. Martín-Ruiz, J.M. Herrerías-
Gutiérrez, and J.M. Esteban-Carretero (1999). Are there changes in gastric emptying
during the menstrual cycle? Scand. J. Gastroenterol. 34:772-776.
Cadarette, B.S., M.N. Sawka, M.M. Toner, and K.B. Pandolf (1984). Aerobic fitness and the
hypohydration response to exercise-heat stress. Aviat. Space Environ. Med. 55:507-
512.
Calzone, W.L., C. Silva, D.L. Keefe, and N.S. Stachenfeld (2001). Progesterone does not alter
osmotic regulation of AVP. Am. J. Physiol. 281:R2011-2020.
Casa, D.J., R.L. Stearns, R.M. Lopez, M.S. Ganio, B.P. McDermott, S. Walker Yeargin, L.M.
Yamamoto, S.M. Mazerolle, M.W. Roti, L.E. Armstrong, and C.M. Maresh (2010).
Influence of hydration on physiological function and performance during trail running in
the heat. J. Athl. Train. 45:147-156.
Chapelle, L., B. Tassignon, N. Rommers, E. Mertens, P. Mullie, and P. Clarys (2020). Preexercise
hypohydration prevalence in soccer players: A quantitative systematic review.
Eur. J. Sport Sci. 20:744-755.
Cheuvront, S.N., R. Carter, 3rd, J.W. Castellani, and M.N. Sawka (2005). Hypohydration
impairs endurance exercise performance in temperate but not cold air. J. Appl. Physiol.
99:1972-1976.
Cheuvront, S.N., and R.W. Kenefick (2014). Dehydration: physiology, assessment, and
performance effects. Compr. Physiol. 4: 257-285.
Courtenay, W. (2011). Dying to be men: Psychosocial, environmental, and biobehavioral
directions in promoting the health of men and boys. 1st Edition. Routledge. 526 pages.
D'Anci K, E., A. Vibhakar, J.H. Kanter, C.R. Mahoney, and H.A. Taylor (2009). Voluntary
dehydration and cognitive performance in trained college athletes. Percept. Mot. Skills
109:251-269.
Driscoll, R.L., D.G. McCarthy, M.S. Palmer, and L.L. Spriet (2020). Mild dehydration impaired
intermittent sprint performance and thermoregulation in females. Appl. Physiol. Nutr.
Metab. 45:1045-1048.
Evans, G.H., S.M. Shirreffs, and R.J. Maughan (2011). The effects of repeated ingestion of
high and low glucose-electrolyte solutions on gastric emptying and blood 2H2O
concentration after an overnight fast. Br. J. Nutr. 106:1732-1739.
Freemas, J.A., Goss, C.S., Ables, R., Baker, T.B., Bruinvels, G., Mündel, T., Martin, B.J.,
Carter, S.J., Chapman, R.F., Schlader, Z.J. Fluid balance during physical work in the
heat is not modified by the menstrual cycle when fluids are freely available. J Appl
Physiol 2023. Online ahead of print.
Gagnon, D., and G.P. Kenny (2012). Does sex have an independent effect on thermoeffector
responses during exercise in the heat? J. Physiol. 590:5963-5973.
Gagnon, D., O. Jay, and G.P. Kenny (2013). The evaporative requirement for heat balance
determines whole-body sweat rate during exercise under conditions permitting full
evaporation. J. Physiol. 591:2925-2935.
Gann, J.J., T.L. Andre, A.R. Gallucci, and D.S. Willoughby (2021). Effects of hypohydration
on muscular strength, endurance, and power in women. J. Strength Cond. Res.
35(Suppl 1):S102-S106.
Giersch, G.E.W., M.C. Morrissey, R.K. Katch, A.T. Colburn, S.T. Sims, N.S. Stachenfeld, and
D.J. Casa (2020). Menstrual cycle and thermoregulation during exercise in the heat: A
systematic review and meta-analysis. J. Sci. Med. Sport. 23:1134-1140.
Giersch, G.E.W., M.C. Morrissey, C.R. Butler, A.T. Colburn, Z.S. Demarais, S.A. Kavouras, O.
Jay, N. Charkoudian, and D.J. Casa (2021). Sex difference in initial thermoregulatory
response to dehydrated exercise in the heat. Physiol. Rep. 9:e14947.
Gifford, R.M., T. Todisco, M. Stacey, T. Fujisawa, M. Allerhand, D.R. Woods, and R.M.
Reynolds (2019). Risk of heat illness in men and women: A systematic review and
meta-analysis. Environ. Res. 171:24-35.
Gill, R.C., P.D. Murphy, H.R. Hooper, K.L. Bowes, and Y.J. Kingma (1987). Effect of the
menstrual cycle on gastric emptying. Digestion 36:168-174.
Gonzalez-Alonso, J., J.A. Calbet, and B. Nielsen (1998). Muscle blood flow is reduced with
dehydration during prolonged exercise in humans. J. Physiol. 513:895-905.
Goulet, E.D. (2011). Effect of exercise-induced dehydration on time-trial exercise
performance: a meta-analysis. Br. J. Sports Med. 45:1149-1156.
Gutierrez, A., J.L. Mesa, J.R. Ruiz, L.J. Chirosa, and M.J. Castillo (2003). Sauna-induced
rapid weight loss decreases explosive power in women but not in men. Int. J. Sports
Med. 24:518-522.
Hellmig, S., F. Von Schoning, C. Gadow, S. Katsoulis, J. Hedderich, U.R. Folsch, and E.
Stuber (2006). Gastric emptying time of fluids and solids in healthy subjects determined
by 13C breath tests: influence of age, sex and body mass index. J. Gastroenterol.
Hepatol. 21:1832-1838.
Hew, T.D. (2005). Women hydrate more than men during a marathon race: hyponatremia in
the Houston marathon: a report on 60 cases. Clin. J. Sport Med. 15:148-153.
Hoffman, J.R., D.R. Williams, N.S. Emerson, M.W. Hoffman, A.J. Wells, D.M. McVeigh, W.P.
McCormack, G.T. Mangine, A.M. Gonzalez, and M.S. Fragala (2012). L-alanyl-Lglutamine
ingestion maintains performance during a competitive basketball game. J.
Int. Soc. Sports Nutr. 9:4.
Holland, J.J., T.L. Skinner, C.G. Irwin, M.D. Leveritt, and E.D.B. Goulet (2017). The influence
of drinking fluid on endurance cycling performance: a meta-analysis. Sports Med.
47:2269-2284.
Hooton, T.M., M. Vecchio, A. Iroz, I. Tack, Q Dornic, I. Seksek, and Y. Lotan (2018). Effect of
increased daily water intake in premenopausal women with recurrent urinary tract
infections: A randomized clinical trial. J. Am. Med Assoc. Intern. Med. 178:1509-1515.
Horowitz, M., G.J. Maddern, B.E. Chatterton, P.J. Collins, O.M. Petrucco, R. Seamark, and
D.J. Shearman (1985). The normal menstrual cycle has no effect on gastric emptying.
Br. J. Obstet. Gynaecol. 92:743-746.
Jay, O. (2014). Unravelling the true influences of fitness and sex on sweating during exercise.
Exp. Physiol. 99:1265-1266.
Kavouras, S.A. (2019). Hydration, dehydration, underhydration, optimal hydration: are we
barking up the wrong tree? Eur. J. Nutr. 58:471-473.
Kenney, W.L. (1985). A review of comparative responses of men and women to heat stress.
Environ. Res. 37:1-11.
Kolka, M.A., and L.A. Stephenson (1989). Control of sweating during the human menstrual
cycle. Eur. J. Appl. Physiol. 58:890-895.
Kolka, M.A., and L.A. Stephenson (1997). Interaction of menstrual cycle phase, clothing
resistance and exercise on thermoregulation in women. J. Therm. Biol. 22:137-141.
Kozlowski, S., and B. Saltin (1964). Effect of sweat loss on body fluids. J. Appl. Physiol.
19:1119-1124.
Kuwahara, T., Y. Inoue, M. Abe, Y. Sato, and N. Kondo (2005). Effects of menstrual cycle and
physical training on heat loss responses during dynamic exercise at moderate intensity
in a temperate environment. Am. J. Physiol. Regul. 288:R1347-R1353.
Leiper, J.B. (2015). Fate of ingested fluids: factors affecting gastric emptying and intestinal
absorption of beverages in humans. Nutr. Rev. 73(Suppl 2):57-72.
Logan-Sprenger, H.M., G.J. Heigenhauser, K.J. Killian, and L.L. Spriet (2012). Effects of
dehydration during cycling on skeletal muscle metabolism in females. Med. Sci. Sports
Exerc. 44:1949-1957.
MacLeod, H., and C. Sunderland (2009). Fluid balance and hydration habits of elite female
field hockey players during consecutive international matches. J. Strength Cond. Res.
23:1245-1251.
MacLeod, H., and C. Sunderland (2012). Previous-day hypohydration impairs skill
performance in elite female field hockey players. Scand. J. Med. Sci. Sports 22:430-
438.
Maughan, R.J., M. McArthur, and S.M. Shirreffs (1996). Influence of menstrual status on
fluid replacement after exercise induced dehydration in healthy young women. Br. J.
Sports Med. 30:41-47.
McDermott, B.P., S.A. Anderson, L.E. Armstrong, D.J. Casa, S.N. Cheuvront, L. Cooper, W.L.
Kenney, F.G. O'Connor, and W.O. Roberts (2017). National Athletic Trainers' Association
position statement: fluid replacement for the physically active. J. Athl. Train. 52:877-895.
Meyer, F., O. Bar-Or, D. MacDougall, and G.J. Heigenhauser (1992). Sweat electrolyte loss
during exercise in the heat: effects of gender and maturation. Med. Sci. Sports Exerc.
24:776-781.
Sports Science Exchange (2023) Vol. 36, No. 237, 1- 10
9
Montain, S.J., and E.F. Coyle (1992). Influence of graded dehydration on hyperthermia and
cardiovascular drift during exercise. J. Appl. Physiol. 73:1340-1350.
Montain, S.J., S.A. Smith, R.P. Mattot, G.P. Zientara, F.A. Jolesz, and M.N. Sawka (1998).
Hypohydration effects on skeletal muscle performance and metabolism: a 31P-MRS
study. J. Appl. Physiol. 84:1889-1894.
Munoz, C.X., E.C. Johnson, A.L. McKenzie, I. Guelinckx, G. Graverholt, D.J. Casa, C.M.
Maresh, and L.E. Armstrong (2015). Habitual total water intake and dimensions of
mood in healthy young women. Appetite 92:81-86.
Notley, S.R., S. Dervis, M.P. Poirier, and G.P. Kenny (2019). Menstrual cycle phase does not
modulate whole body heat loss during exercise in hot, dry conditions. J. Appl. Physiol.
126:286-293.
Nuccio, R.P., K.A. Barnes, J.M. Carter, and L.B Baker. (2017). Fluid balance in team sport
athletes and the effect of hypohydration on cognitive, technical, and physical
performance. Sports Med. 47:1951-1982.
Nygaard, I., and M. Linder (1997). Thirst at work--an occupational hazard? Int. Urogynecol.
J. Pelvic Floor Dysfunct. 8:340-343.
Periard, J.D., S. Racinais, T. Timpka, O. Dahlstrom, A. Spreco, J. Jacobsson, V. Bargoria, K.
Halje, and J.M. Alonso (2017). Strategies and factors associated with preparing for
competing in the heat: a cohort study at the 2015 IAAF World Athletics Championships.
Br. J. Sports Med. 51:264-270.
Perrier, E.T. (2017). Shifting focus: From hydration for performance to hydration for health.
Ann. Nutr. Metab. 70(Suppl 1):4-12.
Pross, N., A. Demazieres, N. Girard, R. Barnouin, F. Santoro, E. Chevillotte, A. Klein, and L.
Le Bellego (2013). Influence of progressive fluid restriction on mood and physiological
markers of dehydration in women. Br. J. Nutr. 109:313-321.
Quinkler, M., B. Meyer, C. Bumke-Vogt, C. Grossmann, U. Gruber, W. Oelkers, S. Diederich,
and V. Bahr (2002). Agonistic and antagonistic properties of progesterone metabolites
at the human mineralocorticoid receptor. Eur. J. Endocrinol. 146:789-799.
Rehrer, N.J., G.M. Janssen, F. Brouns, and W.H. Saris (1989). Fluid intake and gastrointestinal
problems in runners competing in a 25-km race and a marathon. Int. J. Sports Med.
10(Suppl 1):S22-S25.
Rodriguez-Giustiniani, P., and S.D.R. Galloway (2019). Influence of peak menstrual cycle
hormonal changes on restoration of fluid balance after induced dehydration. Int. J.
Sport Nutr. Exerc. Metab. 29:651-657.
Rodriguez-Giustiniani, P., N. Rodriguez-Sanchez, and S.D.R. Galloway (2022). Fluid and
electrolyte balance considerations for female athletes. Eur. J. Sport Sci. 22:697-708.
Savoie, F.A., R.W. Kenefick, B.R. Ely, S.N. Cheuvront, and E.D. Goulet (2015). Effect of
hypohydration on muscle endurance, strength, anaerobic power and capacity and
vertical jumping ability: A meta-analysis. Sports Med. 45:1207-1227.
Sawka, M.N., M.M. Toner, R.P. Francesconi, and K.B. Pandolf (1983). Hypohydration and
exercise: effects of heat acclimation, gender, and environment. J. Appl. Physiol.
55:1147-1153.
Sawka, M.N., A.Y. Young, R.P. Francesconi, S.R. Muza, and K.B. Pandolf (1985).
Thermoregulatory and blood responses during exercise at graded hypohydration levels.
J. Appl. Physiol. 59:1394-1401.
Sawka, M.N., L.M. Burke, E.R, Eichner, R.J. Maughan, S.J. Montain, and N.S. Stachenfeld
(2007). American College of Sports Medicine position stand. Exercise and fluid
replacement. Med. Sci. Sports Exerc. 39:377-390.
Shapiro, Y., K.B. Pandolf, B.A. Avellini, N.A. Pimental, and R.F. Goldman (1980). Physiological
responses of men and women to humid and dry heat. J. Appl. Physiol. 49:1-8.
Shi, X., M.K. Horn, K.L. Osterberg, J.R. Stofan, J.J. Zachwieja, C.A. Horswill, D.H. Passe,
and R. Murray (2004). Gastrointestinal discomfort during intermittent high-intensity
exercise: effect of carbohydrate-electrolyte beverage. Int. J. Sport Nutr. Exerc. Metab.
14:673-683.
Smith, C.J., and G. Havenith (2012). Body mapping of sweating patterns in athletes: a sex
comparison. Med. Sci. Sports Exerc. 44: 2350-2361.
Sollanek, K.J., M. Tsurumoto, S. Vidyasagar, R.W. Kenefick, and S.N. Cheuvront (2018).
Neither body mass nor sex influences beverage hydration index outcomes during
randomized trial when comparing 3 commercial beverages. Am. J. Clin. Nutr. 107:544-
549.
Soo, K., and G. Naughton (2007). The hydration profile of female cricket players during
competition. Int. J. Sport Nutr. Exerc. Metab. 17:14-26.
Stachenfeld, N.S. (2014). Sodium ingestion, thirst and drinking during endurance exercise.
Sports Science Exchange. #122.
Stachenfeld, N.S., and H.S. Taylor (2009). Sex hormone effects on body fluid and sodium
regulation in women with and without exercise-associated hyponatremia. J. Appl.
Physiol. 107:864-872.
Stachenfeld, N.S., C. Silva, D.L. Keefe, C.A. Kokoszka, and E.R. Nadel (1999). Effects of oral
contraceptives on body fluid regulation. J. Appl. Physiol. 87:1016-1025.
Stachenfeld, N.S., C.A. Leone, E.S. Mitchell, E. Freese, and L. Harkness. (2018). Water
intake reverses dehydration associated impaired executive function in healthy young
women. Physiol. Behav. 185:103-111.
Stephenson, L.A., and M.A. Kolka (1985). Menstrual cycle phase and time of day alter
reference signal controlling arm blood flow and sweating. Am. J. Physiol.
249:R186-R191.
Suh, H., H.R. Lieberman, L.T. Jansen, A.T. Colburn, J.D. Adams, A.D. Seal, C.L. Butts, T.M.
Kirkland, O. Melander, T. Vanhaecke, A. Dolci, G. Lemetais, E.T. Perrier, and S.A.
Kavouras (2021). Cellular dehydration acutely degrades mood mainly in women: a
counterbalanced, crossover trial. Br. J. Nutr. 125:1092-1100.
Szinnai, G., H. Schachinger, M.J. Arnaud, L. Linder, and U. Keller, U. (2005). Effect of water
deprivation on cognitive-motor performance in healthy men and women. Am. J. Physiol.
289:R275-R280.
Thigpen, L.K., J.M. Green, and E.K. O'Neal (2014). Hydration profile and sweat loss
perception of male and female division II basketball players during practice. J. Strength
Cond. Res. 28:3425-3431.
Visser, M., D. Gallagher, P. Deurenberg, J. Wang, R.N. Pierson, Jr., and S.B. Heymsfield
(1997). Density of fat-free body mass: relationship with race, age, and level of body
fatness. Am. J. Physiol, 272:E781-E787.
Vokes, T.J., N.M. Weiss, J. Schreiber, M.B. Gaskill, and G.L. Robertson (1988).
Osmoregulation of thirst and vasopressin during normal menstrual cycle. Am. J.
Physiol. 254:R641-647.
Volpe, S.L., K.A. Poule, and E.G. Bland (2009). Estimation of prepractice hydration status of
National Collegiate Athletic Association Division I athletes. J. Athl. Train. 44:624-629.
Vyas, S., D. Varshney, P. Sharma, R. Juyal, V. Nautiyal, and V. Shrotriya (2015). An overview
of the predictors of symptomatic urinary tract infection among nursing students. Ann.
Med. Health Sci. Res. 5:54-58.
Watanabe, K., E.J. Stohr, K. Akiyama, S. Watanabe, and J. Gonzalez-Alonso (2020).
Dehydration reduces stroke volume and cardiac output during exercise because of
impaired cardiac filling and venous return, not left ventricular function. Physiol. Rep.
8:e14433.
Wickham, K.A., D,G, McCarthy, L.L. Spriet, and S.S. Cheung (2021). Sex differences in the
physiological responses to exercise-induced dehydration: consequences and
mechanisms. J. Appl. Physiol. 131:504-510.
Yanovich, R., I. Ketko, and N. Charkoudian (2020). Sex differences in human thermoregulation:
Relevance for 2020 and beyond. Physiology 35:177-184