KEY POINTS
- Replacement of fluid losses during/after exercise is a process involving several steps, starting with fluid ingestion, followed by gastric emptying, and culminating in fluid absorption into the bloodstream and distribution to the intra- and extracellular fluid spaces of the body.
- Adequate fluid replacement is influenced in part by beverage composition, as the presence of certain ingredients (and the types/amounts) can facilitate or hinder the rehydration process.
- Beverage palatability has a substantial impact on voluntary fluid intake. In general, cool (~15°C) fluids with flavoring, electrolytes, and light sweetening are preferred by athletes and lead to increased fluid intake.
- The presence of sodium in a beverage can promote fluid intake (thirst and drink palatability), whole-body fluid retention (renal water reabsorption), and fluid distribution into the extracellular space (plasma volume maintenance).
- A small amount of carbohydrate (e.g., 1 – 3%) in a sports drink promotes intestinal fluid absorption. Highly concentrated carbohydrate drinks (≥ 8%) can delay fluid delivery. However, formulating with multiple transportable carbohydrates (e.g., glucose and fructose) can increase the rate of gastric emptying and intestinal fluid absorption of beverages with high carbohydrate concentrations.
- During post-exercise rehydration, slowing the appearance of the ingested fluid into the circulation via increased beverage energy density (e.g., fluids with carbohydrate, protein; or drinking water with meals) attenuates diuresis and promotes fluid retention.
INTRODUCTION
Substantial volumes of fluid can be lost during intense or prolonged exercise as a consequence of thermoregulatory sweating (Barnes et al., 2019). In these situations, athletes need to drink during/after exercise to replace fluids lost to avoid potential negative effects of dehydration (McDermott et al., 2017). As illustrated in Figure 1, fluid replacement is a multi-step process involving a number of physiological systems. The process includes fluid intake, fluid delivery to the bloodstream (via gastric emptying and intestinal absorption), distribution within the body fluid compartments (e.g., for maintenance of plasma volume), and whole-body fluid retention (via renal water reabsorption). Adequate fluid replacement is influenced by beverage composition, as certain ingredients can facilitate or hinder one or more steps in the rehydration process (Baker & Jeukendrup, 2014). The purpose of this Sports Science Exchange (SSE) article is to provide an overview of the scientific principles of fluid replacement and discuss the role of beverage formulation (carbohydrates, electrolytes, flavor, and other ingredients or factors) on the rehydration process.
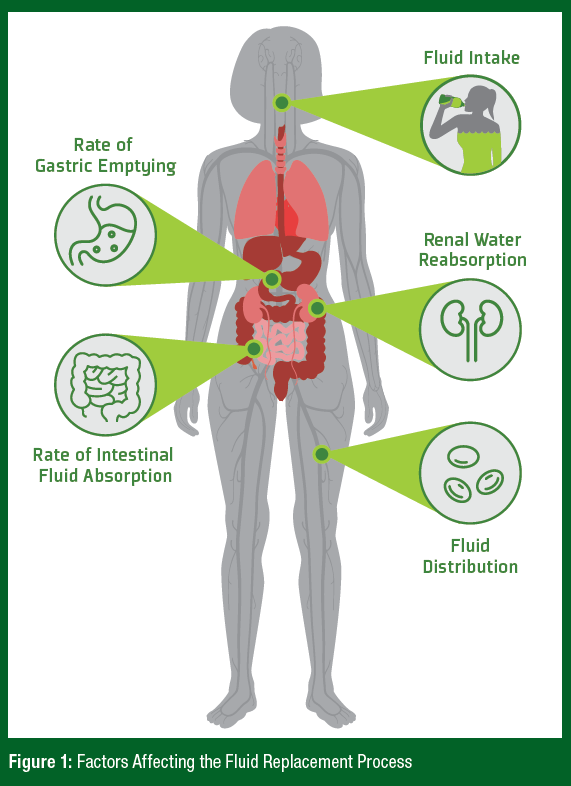
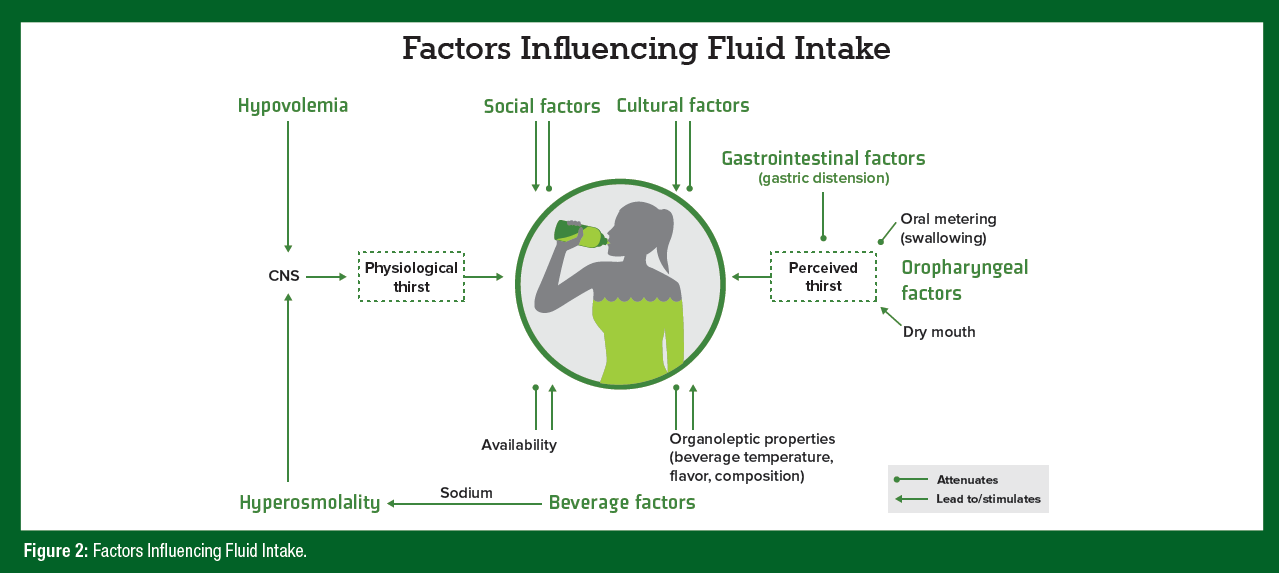
FLUID INTAKE
The first step in rehydration is the act of drinking water or other fluids. This may sound simple, but as shown in Figure 2, fluid intake is actually very complex and dictated by multiple mechanisms (Adolph et al., 1954). Physiological (or regulatory) thirst is stimulated in response to cellular dehydration (hyperosmolality) and extracellular hypohydration (hypovolemia). However, ad libitum or voluntary fluid intake is also influenced by a complex interaction of numerous other non-regulatory factors. These include perceived thirst, social influences, cultural preferences, and beverage-related factors (Baker & Jeukendrup, 2014; Passe, 2001). As discussed in the next section, beverage composition can impact fluid intake through its effects on physiological thirst (e.g., through sodium intake) as well as drink palatability (e.g., through organoleptic properties such as flavor, sweetness, saltiness, and temperature).
Beverage Palatability
Flavoring, sweetness, and electrolytes can help improve beverage palatability (i.e., liking and acceptability) and increase ad libitum fluid intake during exercise compared with water. This has been demonstrated in youth and adults, male and female subjects, and in the field during training sessions as well as in controlled laboratory conditions (Minehan et al., 2002; Passe et al., 2000; 2004; Rivera-Brown et al., 1999; Wilk & Bar-Or, 1996). For example, during intermittent exercise in the heat 9 – 12-year-old boys’ fluid intake was highest with a grape-flavored 6% carbohydrate-electrolyte drink, followed by grape-flavored water, then unflavored water. The boys experienced mild hypohydration with water but maintained euhydration when flavor and/or sweetness/ electrolytes were added to the water (Wilk & Bar-Or, 1996). Similarly, in a study with junior elite male and female netball and basketball athletes, better fluid balance was achieved with flavored drinks (1% and 6.8% carbohydrate-electrolyte solutions) compared with water during training sessions (Minehan et al., 2002).
While hedonic ratings are typically higher for flavored/sweetened drinks, some studies have found minimal impact on ad libitum fluid intake (Rivera-Brown et al., 2008; Taim et al., 2021). In addition, some studies have found that ad libitum intake of unflavored water was sufficient to replace sweat losses during exercise (Baker et al., 2005; Wilk et al., 2007). The inconsistency in results could be due to various factors related to study design, exercise and environmental conditions, among other factors. It is also important to note that the perception and acceptability of beverage flavor, sweetness, tartness, mouthfeel, and aftertaste are modified by physical activity (Murray & Stofan, 2001), as certain flavors or attributes may be more acceptable during exercise than at rest (Passe et al., 2000).
Beverage temperature also has a substantial impact on palatability and voluntary fluid intake. During exercise in temperate to hot conditions palatability and fluid intake seem to be maximized when beverage temperature is cool (15°C), followed by cold (0 – 7°C), then temperate (22°C); while warm-hot beverages are the least palatable (32 – 46°C) (Burdon et al., 2012). No studies to the author’s knowledge have investigated fluid temperature, palatability, and fluid intake during exercise in cold environments.
Electrolytes
In addition to their effects on beverage palatability, electrolytes (particularly sodium) stimulate the physiological drive to drink (Nose et al., 1988). This is because ingestion of sodium increases plasma sodium concentration and in turn plasma osmolality. The sensory information from the osmoreceptors regarding plasma tonicity feeds into higher brain regions to stimulate thirst. The presence of low to moderate amounts of sodium (e.g., 18 – 40 mmol/L) improves beverage palatability and increases ad libitum fluid intake (Clapp et al., 2000; Passe, 2001; Wemple et al., 1997). On the other hand, consumption of plain water decreases plasma osmolality and sodium concentration, which reduces the drive to drink (Nose et al., 1988). However, palatability and voluntary fluid intake may decrease with high beverage sodium concentrations (≥ 50 mmol/L) (Passe, 2001; Wemple et al., 1997). In these instances, other anions (such as citrate) could be used in place of chloride (the typical anion with sodium) to decrease beverage saltiness (Shirreffs, 2003).
FLUID DELIVERY – GASTRIC EMPTYING AND INTESTINAL FLUID ABSORPTION
The second major step in rehydration is delivery of fluid into the bloodstream. This process involves both emptying of fluid from the stomach and transport of water across the intestinal epithelium (Figure 3). Gastric emptying of fluids is regulated by the interaction of gastric volume and feedback inhibition related to the nutrient content of the small intestine. As such, gastric emptying can occur at a maximal rate of up to ~15 – 20 mL/min (~1 L/h) by maintaining a high gastric volume with ingestion of either water or a dilute carbohydrate solution (Costill & Saltin, 1974; Lambert et al., 2012).
After leaving the stomach, absorption of water and solutes occurs primarily in the proximal small intestine (duodenum and jejunum). Absorption of solute (e.g., carbohydrate and sodium) from the intestinal lumen occurs by diffusion along electrochemical gradients and by specific transport mechanisms in the brush border membrane of intestinal epithelial cells. On the other hand, water uptake is a passive process, dependent on an osmotic gradient which is created by absorption of solutes – i.e., water follows solute (Murray & Shi, 2006).
The rates of gastric emptying and intestinal fluid absorption are impacted by various types of stressors. For example, ≥ 3% dehydration and heat stress impair gastric emptying (Neufer et al., 1989; Rehrer et al., 1990) and exercise intensities greater than ~70 – 75% VO2max decrease gastric emptying and intestinal water absorption (Costill & Saltin, 1974).
Various aspects of beverage composition can have a significant impact on gastric emptying and/or fluid absorption and will be discussed next.
Carbohydrates
Since water follows solute, the presence of solute (particularly carbohydrate), is important to stimulate water absorption. Accordingly, the World Health Organization’s formulation for oral rehydration solutions (ORS) intended for patients with diarrheal disease includes a small amount of glucose (1.35%) because cotransport of glucose and sodium (via the sodium-glucose link transporter (SGLT1)) accelerates water absorption in the small intestine (Atia & Buchman, 2009). Furthermore, studies with healthy individuals have found that the presence of 1-3% carbohydrate in a sports drink improves the rate of fluid delivery compared with carbohydrate-free solutions (Jeukendrup et al., 2009; Rowlands et al., 2022).
Although carbohydrate plays an important role in stimulating water absorption, the presence of carbohydrate in high concentrations can lead to diminishing returns. In general, beverages with high energy density result in a slower rate of gastric emptying and fluid delivery (Brouns et al., 1995; Evans et al., 2011; Jeukendrup et al., 2009). For example, beverages with ≥ 8% carbohydrate solution have been found to decrease the gastric emptying rate and increase gastrointestinal discomfort compared with water (Murray et al., 1999; Rehrer et al., 1992; Shi et al., 2004). In addition, there is a significant inverse relation between beverage osmolality (which is primarily driven by carbohydrate concentration) and intestinal fluid absorption (Rowlands et al., 2022; Shi & Passe, 2010). However, as discussed in more detail below, formulating with multiple transportable carbohydrates can increase the rate of gastric emptying and intestinal fluid absorption of beverages with high carbohydrate concentrations (Jeukendrup & Moseley, 2010; Shi et al., 1995; 2000).
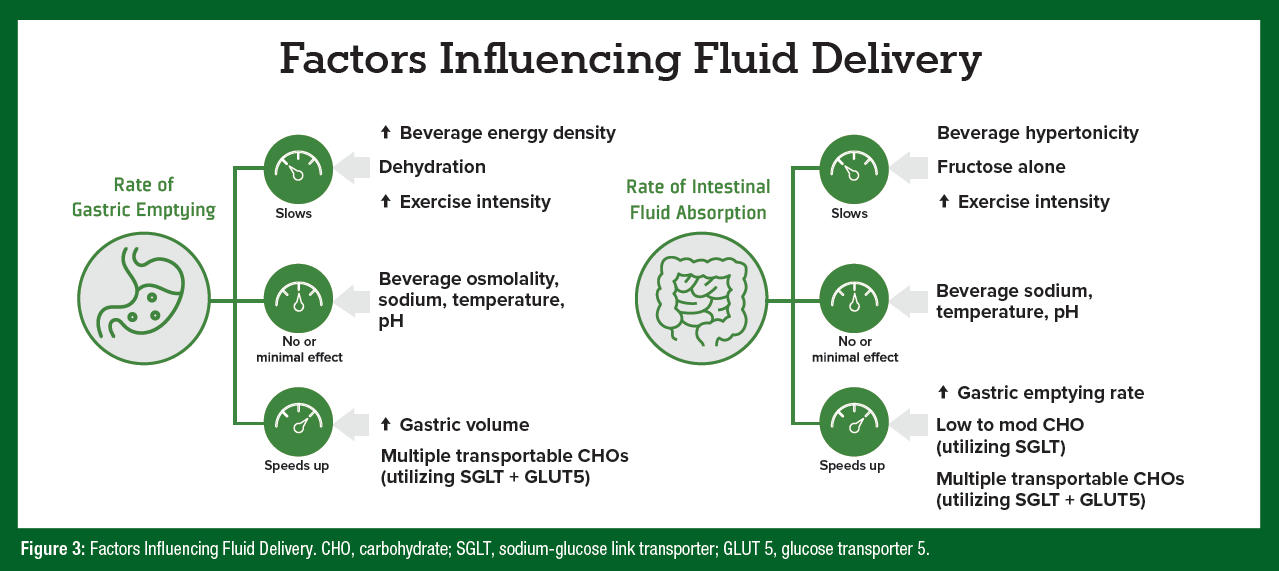
The type of carbohydrate in a sports drink impacts the rate of intestinal fluid and solute absorption because individual carbohydrate types are transported across enterocytes via different mechanisms. For example, a solution containing only glucose (actively transported via SGLT1 transporter) is absorbed faster than a fructose-only (facilitated diffusion via glucose transporter 5 (GLUT-5)) solution. However, a drink with multiple transportable carbohydrates (e.g., glucose and fructose) increases solute and water absorption compared with solutions with a single transportable carbohydrate (Jentjens et al., 2006; Shi & Passe, 2010). Sucrose can either be hydrolyzed into glucose and fructose or utilize its own disaccharide/sucrose transporter (SCRT) (Ferraris & Diamond, 1997). In addition, carbohydrate type significantly impacts the osmolality of a beverage, with maltodextrin having lower osmolality than mono- and disaccharides.
Electrolytes
Although it is well established that sodium is important for SGLT1 transport of substrate and water flux across enterocytes, beverage sodium has been found to play only a minor role in intestinal fluid absorption. This is likely because sodium is readily available through intestinal secretions (Gisolfi et al., 1995). Limited information is available on the effects of other electrolytes on fluid delivery. One study reported faster intestinal absorption when the conjugate anion of sodium was chloride compared with bicarbonate or sulfate (Fordtran, 1975); however, more research is needed for other anion comparisons.
Protein
Protein contributes to the energy density of a beverage and therefore can have a significant impact on the rate of gastric emptying and fluid delivery into circulation. In addition, the clotting of casein in the stomach from milk-based products further delays gastric emptying and slows intestinal fluid absorption compared to energy-matched drinks with whey protein (Calbet & Holst, 2004) or glucose (Burn-Murdoch et al., 1978). While the inclusion of protein reduces the rate of gastric emptying and intestinal fluid absorption, this delay in the delivery of fluid into the circulation may be advantageous for fluid retention during post-exercise rehydration (see Fluid Distribution and Retention section below).
Amino Acids
Amino acids have been investigated for their effects on intestinal water and solute absorption, primarily in the context of oral rehydration from diarrheal disease. This is because amino acids such as glycine, alanine, L-glutamine, and L-arginine are actively transported across the gut (via sodium-coupled transporters separate from SGLT-1), which in turn is thought to increase water flux into the bloodstream (Wapnir et al., 1997). Most studies to date have found no advantage of amino acid-based ORS over glucose-based ORS in rehydrating patients with diarrhea (Bhan et al., 1994; Gutierrez et al., 2007), although this is an area of continued research (Das et al., 2022). Moreover, no studies have measured the efficacy of amino acid-based solutions on intestinal fluid absorption rates in healthy humans during/after exercise. Thus, more research is needed to better understand the effects of amino acids on fluid delivery, especially with respect to rehydration beverages for athletes.
Other Beverage Characteristics
Additional factors that are thought to impact fluid delivery include beverage temperature and pH. Some studies have found significant differences in gastric emptying rates between cold (4 – 5°C) beverages and warm/hot (35 – 50°C) beverages, but only in the first ~5 – 15 min after ingestion. Furthermore, the effect is inconsistent with some studies finding that cold beverages empty faster (Costill & Saltin, 1974) and others reporting faster gastric emptying rates with hot beverages (Lambert & Maughan, 1992). Even so, there is no effect of beverage temperature on overall gastric emptying rates or intestinal fluid absorption. This is likely because intragastric temperature rapidly equilibrates with core body temperature after fluid ingestion. Similarly, the pH of the stomach and intestinal luminal contents are minimally affected by the levels of acidity present in most beverages and thus beverage pH is unlikely to influence fluid delivery (Leiper, 2015).
FLUID DISTRIBUTION AND RETENTION
Once the ingested fluid is absorbed into the circulation, it is distributed to the intracellular and extracellular fluid compartments according to osmotic gradients. The maintenance of extracellular volume, particularly plasma volume, is important for cardiovascular and thermoregulatory function. To maintain plasma volume and overall whole-body fluid balance, it is important that ingested fluid is retained in the body instead of losing it through urination. There are two main ways to attenuate diuresis and promote fluid retention: 1) by stimulating renal water reabsorption (e.g., via sodium) and/or 2) by slowing the appearance of the ingested fluid into the circulation (e.g., via increased beverage energy density) (Baker & Jeukendrup, 2014). Beverage composition can have a significant effect on fluid distribution and fluid retention (Figure 4) during exercise and especially at rest. During moderate to heavy dynamic exercise, urine output decreases by 50 – 70% due to decreases in the glomerular filtration rate and renal blood flow mediated by an increase in renal vasoconstriction (Zambraski, 2012). Thus, most studies measuring the effects of beverage composition on fluid retention are conducted before or after exercise.
Before exercise, the goal is to begin the workout in a euhydrated state. After exercise in which the athlete has incurred a body mass deficit, the goal is to drink to replace sweat losses and restore body fluid balance. Rapid and complete rehydration after exercise is important if the athlete is participating in a practice session or game within the same day or in < 24 h. When there are ≥ 24 h between exercise sessions, adequate fluid can usually be consumed with normal eating and drinking practices (i.e., ad libitum) (Shirreffs & Sawka, 2011).
Electrolytes
The most osmotically active ions in the intracellular and extracellular fluid compartments are potassium and sodium, respectively. Since
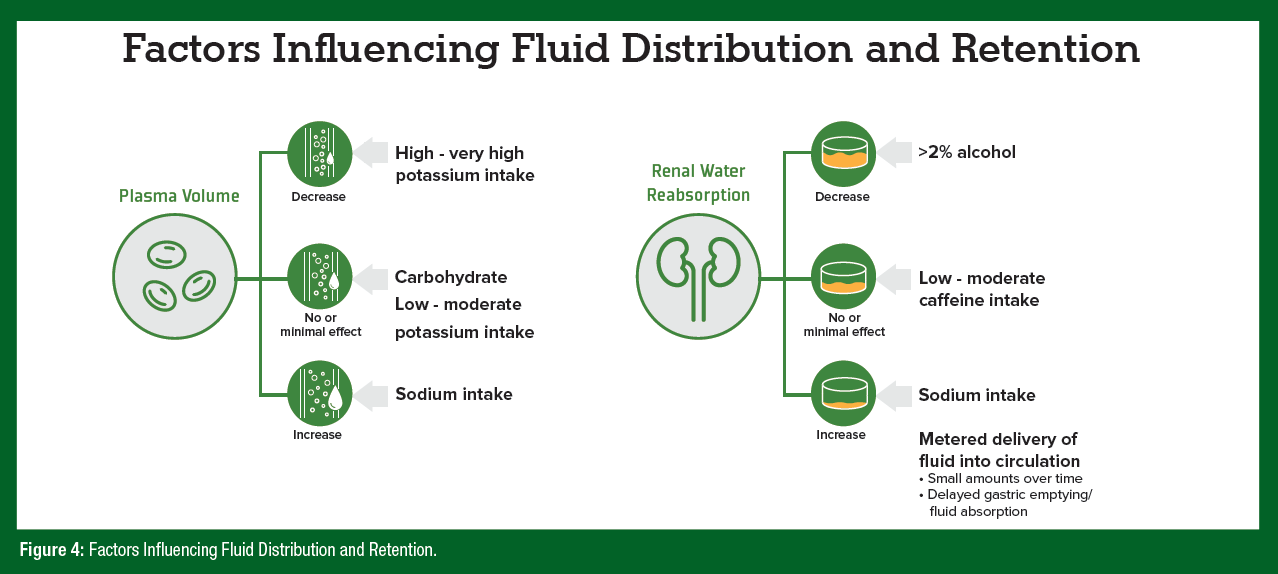
extracellular fluid is the precursor for sweat gland secretions, sweat is comprised mostly of salt water (sodium and chloride concentrations of ~10 – 90 mmol/L) while potassium concentrations are much lower (~2 – 6 mmol/L) (Barnes et al., 2019). Sodium and potassium are often included in sports drinks to help replace losses due to sweating. However, there are some differences in how these two electrolytes may affect fluid distribution and fluid retention.
There is no debate that inclusion of sodium can have a significant impact on fluid distribution and fluid retention. The increase in blood sodium concentration and osmolality with sodium ingestion stimulates renal water reabsorption and thus decreases urine output. Most studies suggest that fluid retention is significantly improved by drinking a beverage with a sodium concentration of ≥ ~20 – 30 mmol/L compared with no or very low sodium drinks (Gonzalez-Alonso et al., 1992; Maughan & Leiper, 1995; Maughan et al., 2019; Perez-Idarraga & Aragon-Vargas, 2014). Furthermore, some studies have found a direct linear relation between beverage sodium concentration and fluid retention (Maughan & Leiper, 1995; Shirreffs & Maughan, 1998). For example, Maughan and Leiper (1995) found that the percentage of fluid retained increased progressively as beverage sodium concentration increased from 2 mmol/L to 26 mmol/L to 52 mmol/L to 100 mmol/L. In addition, because sodium is the primary extracellular cation, ingesting a beverage with sodium promotes fluid retention in the vascular space, which can lead to better plasma volume maintenance or restoration during or after exercise (Gonzalez- Alonso et al., 1992; Hamouti et al., 2014).
Since potassium is an intracellular osmolyte it is thought that its ingestion can promote overall water retention by regulating the level of intracellular fluid. However, studies investigating the effects of potassium-containing drinks on fluid retention have found mixed results. For example, Maughan et al. (1994) reported that ingestion of a 25 mmol/L potassium drink led to lower urine output during postexercise rehydration compared with an electrolyte-free control drink. However, Shirreffs et al. (2007) found no difference in postexercise rehydration between a 30 mmol/L potassium drink and mineral water. Fresh coconut water, which is naturally high in potassium (~50 mmol/L), has also been studied for its effect on post-exercise fluid retention. Again, results have been inconsistent, with about half of the studies showing that coconut water results in better fluid retention while the other half reported no difference in comparison with plain water (Aragon-Vargas, 2016).
Importantly, several studies have shown that the rate of recovery of the plasma volume during post-exercise rehydration is slower with sodium-free, high-potassium drinks (25 – 51 mmol/L) than control drinks. This observation suggests that restoration of intracellular volume occurs at the expense of the extracellular space when high potassium-containing drinks are consumed without sodium (Maughan et al., 1994; Nielsen et al., 1986; Shirreffs et al., 2007). A slower restoration of plasma volume could have significant cardiovascular/thermoregulatory implications for athletes whose next practice or competition is within the same day, especially if exercising in a hot environment.
Carbohydrates
Fluid replacement beverages for athletes often include carbohydrates to top off energy supplies before exercise or help replenish glycogen stores after exercise. Several studies published in the past decade suggest that carbohydrate can also help promote fluid retention. Specifically, carbohydrate solutions ranging from 6% to 12% have been shown to promote greater fluid retention compared with electrolyte-matched, carbohydrate-free placebo solutions in a rested euhydrated state (Maughan et al., 2019) as well as post-exercise rehydration (Evans et al., 2009; Kamijo et al., 2012; Osterberg et al., 2010).
Highly concentrated carbohydrate solutions (e.g., 10 – 12%) could affect fluid retention by delaying gastric emptying or intestinal absorption (from the increased energy density and/or osmolality of the higher carbohydrate beverages), which would effectively delay the appearance of fluid in circulation. The delayed absorption of fluid and/or the higher plasma osmolality elicited by the carbohydrate drink would attenuate renal water excretion (Baker & Jeukendrup, 2014). Other proposed mechanisms, particularly regarding the fluid retention benefits of less concentrated carbohydrate solutions (e.g., 6%), include an insulin-mediated increase in renal sodium and water reabsorption (Kamijo et al., 2012). However, more work is needed to elucidate the exact mechanisms involved and their relative contributions to enhanced fluid retention.
Protein
Protein is often included in post-exercise fluid-replacement beverages to promote muscle protein synthesis and thus aid in the recovery process after physical activity. Research suggests that protein, especially milk protein, may also play a role in enhancing fluid retention in euhydrated individuals at rest (Maughan et al., 2016) or during post-exercise rehydration (Evans et al., 2017). For instance, multiple studies have reported enhanced fluid retention with drinks containing milk protein and carbohydrate compared with energy-matched carbohydrate-only drinks (James et al., 2019). Similarly, Seifert et al. (2006) found greater fluid retention with the addition of 1.5% whey protein to a 6% carbohydrate-electrolyte drink. However, other studies have reported no effect of whey protein on fluid retention during post-exercise rehydration (Evans et al., 2017). The inconsistent findings with the whey protein studies are unclear but may be related to differences in relative fluid intake volume. Subjects consumed a volume equivalent to 100% of body mass loss in the Seifert study compared with 150% replacement in the other studies, finding no effect of whey protein on fluid retention (Evans et al., 2017). As discussed above, potential mechanisms by which protein-containing drinks may impact fluid retention seem to be related to a slowing of the overall rate of fluid delivery into the circulation. A delay in fluid entering the bloodstream would consequently slow the reduction in serum osmolality and therefore prolong the stimulus for renal water reabsorption (Evans et al., 2017).
SUMMARY & PRACTICAL APPLICATIONS
Replacement of fluid losses during/after exercise is a process involving several steps, starting with fluid ingestion, and culminating in fluid absorption into the bloodstream where it is eventually distributed into the intra- and extracellular fluid spaces of the body. Adequate fluid replacement is influenced in part by beverage composition, as the presence of certain ingredients (and the types/amounts) can facilitate or hinder the rehydration process. Aside from water, the most important ingredients to consider in a rehydration drink are carbohydrates, electrolytes, and flavor. In addition, the timing of fluid intake, as well as other nutritional goals (e.g., energy provision during exercise or protein synthesis after exercise), are important factors to consider when determining the appropriate fluid replacement beverage for athletes.
During Exercise
The availability of palatable/preferred fluids is the first, and perhaps most important, step in the fluid replacement process to ensure adequate rehydration during exercise. While individual preferences may vary, in general cool (~15°C), lightly sweetened fluids with flavoring and electrolytes are preferred by athletes and lead to increased fluid intake.
Sodium is the most osmotically active ion in the extracellular fluid and the main electrolyte lost is sweat. Thus, sodium is the key electrolyte to consume during exercise when the goal is replacement of sweat fluid losses, maintenance of electrolyte balance, and restoration of plasma volume.
The amount and type of carbohydrate in a beverage play very important roles in rehydration during exercise. Furthermore, depending on the athlete’s goal or the environment/exercise conditions, energy provision may also need to be considered.
- When rehydration is the priority, lower carbohydrate and osmolality formulations are preferred for rapid delivery of fluid into the circulation.
- When both energy provision and fluid replacement are important, the optimal beverage would be formulated to deliver nutrients to the body without impeding gastric emptying and intestinal absorption of water.
- However, when sweat rates are low (e.g., cool weather) or high rates of carbohydrate delivery are necessary (e.g., > 2 h of exercise) more concentrated beverages may be acceptable (Jeukendrup, 2010). The reader is referred to other reviews that discuss the idea of potential trade-offs between carbohydrate and fluid ingestion during exercise (Coyle & Montain, 1992).
Before/After Exercise
The two main ways that beverage composition can attenuate diuresis and promote fluid retention are by stimulating renal water reabsorption (e.g., via sodium) and/or by slowing the appearance of the ingested fluid into circulation (e.g., via increased beverage energy density). However, athletes should consider the duration between exercise bouts when deciding the most appropriate composition of a fluid replacement beverage.
- If the athlete is significantly dehydrated and the next training session or game is within a few hours, rapid rehydration is needed. In these situations, athletes should drink sodium-containing fluid (with relatively low energy density) to promote fluid retention and plasma volume restoration without delaying fluid delivery to the circulation. Energy-dense fluids may not be suitable when rapid rehydration is needed, since delayed gastric emptying could cause gastrointestinal distress during subsequent exercise.
- Milk or other energy-dense carbohydrate/protein-based drinks are effective rehydration beverages when there are several hours between exercise bouts. Another practical way to help attenuate diuresis during rehydration is by drinking water in combination with food, if there is sufficient time to digest a snack/meal before the next exercise session (Evans et al., 2017).
Regarding the rehydration efficacy of common beverage components consumed throughout the day, moderate caffeine (up to 400 mg) does not impact overall fluid balance (Maughan et al., 2019). On the other hand, drinks with > 2% alcohol can impair rehydration because they stimulate diuresis (Evans et al., 2017).
The author is employed by the Gatorade Sports Science Institute, a division of PepsiCo R&D. The views expressed are those of the author and do not necessarily reflect the position or policy of PepsiCo, Inc.
REFERENCES
Adolph, E.F., J.P. Barker, and P.A. Hoy (1954). Multiple factors in thirst. Am. J. Physiol, 178:538-562.
Aragon-Vargas, L.F. (2016). Need of other elements. In: F. Meyer, Z. Szygula, & B. Wilk (Eds.). Fluid Balance, Hydration, and Athletic Performance. Taylor and Francis Group, LLC. pp. 397-425.
Atia, A.N., and A.L. Buchman (2009). Oral rehydration solutions in non-cholera diarrhea: a review. Am. J. Gastroenterol. 104:2596-2604.
Baker, L.B., and A.E. Jeukendrup (2014). Optimal composition of fluid-replacement beverages. Compr. Physiol. 4:575-620.
Baker, L.B., T.A. Munce, and W.L. Kenney (2005). Sex differences in voluntary fluid intake by older adults during exercise. Med. Sci. Sports Exerc. 37:789-796.
Barnes, K.A., M.L. Anderson, J.R. Stofan, K.J. Dalrymple, A.J. Reimel, T.J., Roberts, R.K. Randell, C.T. Ungaro, and L.B. Baker (2019). Normative data for sweating rate, sweat sodium concentration, and sweat sodium loss in athletes: An update and analysis by sport. J. Sports Sci. 37:2356-2366.
Bhan, M.K., D. Mahalanabis, O. Fontaine, and N.F. Pierce (1994). Clinical trials of improved oral rehydration salt formulations: a review. Bull. World Health Organ. 72:945-955.
Brouns, F., J. Senden, E.J. Beckers, and W.H. Saris (1995). Osmolarity does not affect the gastric emptying rate of oral rehydration solutions. J. Parenter. Enteral. Nutr. 19:403- 406.
Burdon, C.A., N.A. Johnson, P.G. Chapman, and H.T. O'Connor (2012). Influence of beverage temperature on palatability and fluid ingestion during endurance exercise: a systematic review. Int. J. Sport Nutr. Exerc. Metab. 22:199-211.
Burn-Murdoch, R.A., M.A. Fisher, and J.N. Hunt (1978). The slowing of gastric emptying by proteins in test meals. J. Physiol. 274:477-485.
Calbet, J.A., and J.J. Holst (2004). Gastric emptying, gastric secretion and enterogastrone response after administration of milk proteins or their peptide hydrolysates in humans. Eur. J. Nutr. 43:127-139.
Clapp, A.J., P.A. Bishop, J.F. Smith, and E.R. Mansfield (2000). Effects of carbohydrate-electrolyte content of beverages on voluntary hydration in a simulated industrial environment. AIHAJ, 61:692-699.
Costill, D.L., and B. Saltin (1974). Factors limiting gastric emptying during rest and exercise. J. Appl. Physiol. 37:679-683.
Coyle, E., and S.J. Montain (1992). Carbohydrate and fluid ingestion during exercise: are there trade-offs? Med. Sci. Sports Exerc. 24:671-678.
Das, R., R.A. Sobi, A.A. Sultana, B. Nahar, P.K. Bardhan, L. Luke, O. Fontaine, and T. Ahmed (2022). A double-blind clinical trial to compare the efficacy and safety of a multiple amino acid-based ORS with the standard WHO-ORS in the management of non-cholera acute watery diarrhea in infants and young children: "VS002A" trial protocol. Trials 23:706.
Evans, G.H., S.M. Shirreffs, and R.J. Maughan (2009). Postexercise rehydration in man: the effects of osmolality and carbohydrate content of ingested drinks. Nutrition 25:905- 913.
Evans, G.H., S.M. Shirreffs, and R.J. Maughan (2011). The effects of repeated ingestion of high and low glucose-electrolyte solutions on gastric emptying and blood 2H2O concentration after an overnight fast. Br. J. Nutr. 106:1732-1739.
Evans, G.H., L.J. James, S.M. Shirreffs, and R.J. Maughan (2017). Optimizing the restoration and maintenance of fluid balance after exercise-induced dehydration. J. Appl. Physiol. 122:945-951.
Ferraris, R.P., and J. Diamond (1997). Regulation of intestinal sugar transport. Physiol. Rev. 77:257-302.
Fordtran, J.S. (1975). Stimulation of active and passive sodium absorption by sugars in the human jejunum. J. Clin. Invest. 55:728-737.
Gisolfi, C.V., R.D. Summers, H.P. Schedl, and T.L. Bleiler (1995). Effect of sodium concentration in a carbohydrate-electrolyte solution on intestinal absorption. Med. Sci. Sports Exerc. 27:1414-1420.
Gonzalez-Alonso, J., C.L. Heaps, and E.F. Coyle (1992). Rehydration after exercise with common beverages and water. Int. J. Sports Med. 13 :399-406.
Gutierrez, C., S. Villa, F.R. Mota, and J.J. Calva (2007). Does an L-glutamine-containing, glucose-free, oral rehydration solution reduce stool output and time to rehydrate in children with acute diarrhoea? A double-blind randomized clinical trial. J. Health Popul. Nutr. 25:278-284.
Hamouti, N., V.E. Fernandez-Elias, J.F. Ortega, and R. Mora-Rodriguez (2014). Ingestion of sodium plus water improves cardiovascular function and performance during dehydrating cycling in the heat. Scand. J. Med. Sci. Sports 24:507-518.
James, L.J., E.J. Stevenson, P.L.S. Rumbold, and C.J. Hulston (2019). Cow's milk as a post-exercise recovery drink: implications for performance and health. Eur. J. Sport Sci. 19:40-48.
Jentjens, R.L., K. Underwood, J. Achten, K. Currell, C.H. Mann, and A.E. Jeukendrup (2006). Exogenous carbohydrate oxidation rates are elevated after combined ingestion of glucose and fructose during exercise in the heat. J. Appl. Physiol. 100:807-816.
Jeukendrup, A.E. (2010). Carbohydrate and exercise performance: the role of multiple transportable carbohydrates. Curr. Opin. Clin. Nutr. Metab. Care 13:452-457.
Jeukendrup, A.E., and L. Moseley (2010). Multiple transportable carbohydrates enhance gastric emptying and fluid delivery. Scand. J. Med. Sci. Sports 20:112-121.
Jeukendrup, A.E., K. Currell, J. Clarke, J. Cole, and A.K. Blannin (2009). Effect of beverage glucose and sodium content on fluid delivery. Nutr. Metab. 6:9.
Kamijo, Y., S. Ikegawa, Y. Okada, S. Masuki, K. Okazaki, K. Uchida, M. Sakurai, and H. Nose (2012). Enhanced renal Na+ reabsorption by carbohydrate in beverages during restitution from thermal and exercise-induced dehydration in men. Am. J. Physiol. 303:R824-R833.
Lambert, C.P., and R.J. Maughan (1992). Accumulation in the blood of a deuterium tracer added to hot and cold beverages. Scand. J. Med. Sci. Sports 2:76-78.
Lambert, G.P., X. Shi, and R. Murray (2012). The gastrointestinal system. In: P.A. Farrell, M.J. Joyner, & V.J. Caiozzo (Eds.). ACSM's Advanced Exercise Physiology (2nd ed.). Lippincott Williams & Wilkins. pp. 348-362.
Leiper, J.B. (2015). Fate of ingested fluids: factors affecting gastric emptying and intestinal absorption of beverages in humans. Nutr. Rev. 73(Suppl 2):57-72.
Maughan, R.J., and J.B. Leiper (1995). Sodium intake and post-exercise rehydration in man. Eur. J. Appl. Physiol. 71:311-319.
Maughan, R.J., J.H. Owen, S.M. Shirreffs, and J.B. Leiper (1994). Post-exercise rehydration in man: effects of electrolyte addition to ingested fluids. Eur. J. Appl. Physiol. 69:209- 215.
Maughan, R.J., P. Watson, P.A. Cordery, N.P. Walsh, S.J. Oliver, A. Dolci, N. Rodriguez- Sanchez, and S.D. Galloway (2016). A randomized trial to assess the potential of different beverages to affect hydration status: development of a beverage hydration index. Am. J. Clin. Nutr. 103:717-723.
Maughan, R.J., P. Watson, P.A.A. Cordery, N.P. Walsh, S.J. Oliver, A. Dolci, N. Rodriguez- Sanchez, and S.D.R. Galloway (2019). Sucrose and sodium but not caffeine content influence the retention of beverages in humans under euhydrated conditions. Int. J. Sport Nutr. Exerc. Metab. 29:51-60.
McDermott, B.P., S.A. Anderson, L.E. Armstrong, D.J. Casa, S.N. Cheuvront, L. Cooper, W.L. Kenney, F.G. O'Connor, and W.O. Roberts (2017). National Athletic Trainers' Association position statement: Fluid replacement for the physically active. J. Athl. Train. 52:877- 895.
Minehan, M.R., M.D. Riley, and L.M. Burke (2002). Effect of flavor and awareness of kilojoule content of drinks on preference and fluid balance in team sports. Int. J. Sport Nutr. Exerc. Metab. 12:81-92.
Murray, R., and J.R. Stofan (2001). Formulating carbohydrate-electrolyte drinks for optimal efficacy. In: R.J. Maughan and R. Murray (eds.). Sports Drinks: Basic Science and Practical Aspects. CRC Press.
Murray, B., and X. Shi (2006). The gastrointestinal system. In C. M. Tipton (Ed.), ACSM's Advanced Exercise Physiology, Lippincott Williams & Wilkins. pp. 357-369.
Murray, R., W. Bartoli, J. Stofan, M. Horn, and D. Eddy (1999). A comparison of the gastric emptying characteristics of selected sports drinks. Int. J. Sport Nutr. 9:263-274.
Neufer, P.D., A.J. Young, and M.N. Sawka (1989). Gastric emptying during exercise: effects of heat stress and hypohydration. Eur. J. Appl. Physiol. 58:433-439.
Nielsen, B., G. Sjogaard, J. Ugelvig, B. Knudsen, and B. Dohlmann (1986). Fluid balance in exercise dehydration and rehydration with different glucose-electrolyte drinks. Eur. J. Appl. Physiol. 55:318-325.
Nose, H., G.W. Mack, X.R. Shi, and E.R. Nadel (1988). Role of osmolality and plasma volume during rehydration in humans. J. Appl. Physiol. 65:325-331.
Osterberg, K.L., S.E. Pallardy, R.J. Johnson, and C.A. Horswill (2010). Carbohydrate exerts a mild influence on fluid retention following exercise-induced dehydration. J. Appl.. Physiol. 108:245-250.
Passe, D.H. (2001). Physiological and psychological determinants of fluid intake. In: R.J. Maughan and R. Murray (Eds.). Sports Drinks: Basic Science and Practical Aspects. CRC Press. pp. 45-87.
Passe, D.H., M. Horn, and R. Murray (2000). Impact of beverage acceptability on fluid intake during exercise. Appetite 35:219-229.
Passe, D.H., M. Horn, J. Stofan, and R. Murray (2004). Palatability and voluntary intake of sports beverages, diluted orange juice, and water during exercise. Int. J. Sport Nutr. Exerc. Metab. 14:272-284.
Perez-Idarraga, A., and L.F. Aragon-Vargas (2014). Postexercise rehydration: potassium-rich drinks versus water and a sports drink. Appl. Physiol. Nutr. Metab. 39:1167-1174.
Rehrer, N.J., E.J. Beckers, F. Brouns, F. ten Hoor, and W.H. Saris (1990). Effects of dehydration on gastric emptying and gastrointestinal distress while running. Med. Sci. Sports Exerc. 22:790-795.
Rehrer, N.J., A.J. Wagenmakers, E.J. Beckers, D. Halliday, J.B. Leiper, F. Brouns, R.J. Maughan, K. Westerterp, and W.H. Saris (1992). Gastric emptying, absorption, and carbohydrate oxidation during prolonged exercise. J. Appl. Physiol. 72:468-475.
Rivera-Brown, A.M., R. Gutierrez, J.C. Gutierrez, W.R. Frontera, and O. Bar-Or (1999). Drink composition, voluntary drinking, and fluid balance in exercising, trained, heat-acclimatized boys. J. Appl. Physiol. 86:78-84.
Rivera-Brown, A.M., F.A. Ramirez-Marrero, B. Wilk, and O. Bar-Or (2008). Voluntary drinking and hydration in trained, heat-acclimatized girls exercising in a hot and humid climate. Eur. J. Appl. Physiol. 103:109-116.
Rowlands, D.S., B.H. Kopetschny, and C.E. Badenhorst (2022). The hydrating effects of hypertonic, isotonic and hypotonic sports drinks and waters on central hydration during continuous exercise: A systematic meta-analysis and perspective. Sports Med. 52:349-375.
Seifert, J., J. Harmon, and P. DeClercq (2006). Protein added to a sports drink improves fluid retention. Int. J. Sport Nutr. Exerc. Metab. 16:420-429.
Shi, X., and D.H. Passe (2010). Water and solute absorption from carbohydrate-electrolyte solutions in the human proximal small intestine: a review and statistical analysis. Int. J. Sport Nutr. Exerc. Metab. 20:427-442.
Shi, X., R.W. Summers, H.P. Schedl, S.W. Flanagan, R. Chang, and C.V. Gisolfi (1995). Effects of carbohydrate type and concentration and solution osmolality on water absorption. Med. Sci. Sports Exerc. 27:1607-1615.
Shi, X., W. Bartoli, M. Horn, and R. Murray (2000). Gastric emptying of cold beverages in humans: effect of transportable carbohydrates. Int. J. Sport Nutr. Exerc. Metab. 10:394-403.
Shi, X., M.K. Horn, K.L. Osterberg, J.R. Stofan, J.J. Zachwieja, C.A. Horswill, D.H. Passe, and R. Murray (2004). Gastrointestinal discomfort during intermittent high-intensity exercise: effect of carbohydrate-electrolyte beverage. Int. J. Sport Nutr. Exerc. Metab. 14 :673-683.
Shirreffs, S.M. (2003). The optimal sports drink. Sportmedizin und Sporttraumatologie, 51:25-29.
Shirreffs, S.M., and R.J. Maughan (1998). Volume repletion after exercise-induced volume depletion in humans: replacement of water and sodium losses. Am. J. Physiol. 274:F868-F875.
Shirreffs, S.M., and M.N. Sawka (2011). Fluid and electrolyte needs for training, competition, and recovery. J. Sports Sci. 29(Suppl 1):S39-S46.
Shirreffs, S.M., L.F. Aragon-Vargas, M. Keil, T.D. Love, and S. Phillips. (2007). Rehydration after exercise in the heat: a comparison of 4 commonly used drinks. Int. J. Sport Nutr. Exerc. Metab. 17:244-258.
Taim, B.C., H.T. Suppiah, J. Wee, M. Lee, J.K.W. Lee, and M. Chia (2021). Palatable flavoured fluids without carbohydrates and electrolytes do not enhance voluntary fluid consumption in male collegiate basketball players in the heat. Nutrients 13:4197.
Wapnir, R.A., M.A. Wingertzahn, and S. Teichberg (1997). L-arginine in low concentration improves rat intestinal water and sodium absorption from oral rehydration solutions. Gut 40:602-607.
Wemple, R.D., T.S. Morocco, and G.W. Mack (1997). Influence of sodium replacement on fluid ingestion following exercise-induced dehydration. Int. J. Sport Nutr. 7:104-116.
Wilk, B., and O. Bar-Or (1996). Effect of drink flavor and NaCl on voluntary drinking and hydration in boys exercising in the heat. J. Appl. Physiol. 80:1112-1117.
Wilk, B., A.M. Rivera-Brown, and O. Bar-Or (2007). Voluntary drinking and hydration in non-acclimatized girls exercising in the heat. Eur. J. Appl. Physiol. 101:727-734.
Zambraski, E.J. (2012). The renal system. In: P.A. Farrell, M.J. Joyner, & V.J. Caiozzo (Eds.), ACSM's Advanced Exercise Physiology (2nd ed.). Lippincott Williams & Wilkins. pp. 551-563.