Key Points
- Repeated sprint activity, characteristic of football, results in a net reduction in muscle glycogen concentrations, which has been associated with impaired performance toward the latter stages of a match.
- The daily intake of carbohydrate should be proportionate to estimated fuel cost of the training session or match.
- The ingestion of 2.5 g of carbohydrate/kg BM in the “pre-match meal” three hours prior to exercise will “top up” stores of glycogen in the muscle and liver.
- The ingestion of 60 g of carbohydrate/h, prior to and during exercise (including halftime) will preserve endogenous glycogen and increase blood glucose concentrations. As a result, players are able to maintain high intensity running throughout the match, identified as key performance attributes of top level football players and teams.
- Muscle damage as a consequence of frequent changes in direction, decelerations from sprints and contact between players during match play can impair glycogen synthesis after exercise.
- Evidence is accumulating that carbohydrate ingestion also has a beneficial impact on skill performance, however future studies are required to determine the exact mechanisms involved.
Introduction
Football performance is characterised by bursts of very intense activity interspersed with periods of recovery at relatively low exercise intensities (Bansgbo, 2014). Carbohydrate and fat are the fuels which provide the player with the energy required for training and matches. The relative contribution of these fuels during exercise will depend on several factors, including the pre-exercise carbohydrate stores, the exercise intensity and duration, and the training status of the player (Jeukendrup, 2003). Nevertheless, high intensity activities in football, sprints, jumps, etc., are supported by anaerobic energy provision. In a 6-s sprint, glycogen will contribute approximately 50% to ATP turnover within the muscle (Cheetham et al., 1986). Thus, repeated sprint activity results in a net reduction in muscle glycogen concentrations. Low muscle glycogen concentrations have been associated with impaired performance, as measured by distance covered at high-intensity toward the latter stages of a match (Bendiksen et al., 2012). The analysis of top level football has revealed the ability to maintain high-intensity running and levels of skill proficiency, especially in the final stages of a match, are key attributes of a top class player and successful team (Mohr et al., 2003). Thus, the preservation of muscle glycogen and blood glucose concentrations may be important in supporting the physical demands of football and also other factors which contribute to football performance such as agility, timing, skills and decision making. Although the exact mechanisms are still to be determined, carbohydrate ingestion before and during intermittent running can delay fatigue and improve performance. To this end, the current review will discuss those studies which have investigated the ingestion of carbohydrate in preparation for, participation in and recovery from football specific exercise.
Glycogen
The importance of muscle glycogen to football performance was first identified in the early 1970s (Saltin, 1973). In this seminal study, quadriceps femoris from recreational players were biopsied at the beginning, halftime and end of a football match. Following the analysis of muscle glycogen content, the authors reported that concentrations were significantly lower on completion of the match (pre: 96 mmol/kg w.w.; halftime: 32 mmol/kg w.w.; end: 9 mmol/kg w.w.). Those players who began the match with low muscle glycogen (45 mmol/kg w.w.) had almost depleted stores by half-time. It was also observed that players who began the match with high muscle glycogen covered a greater distance and spent more of the total time completing high-intensity runs (27% versus 15%) in comparison to those players who began the game with low muscle glycogen.
Over the last four decades, the development and use of new technologies, such as video filming and GPS has allowed the physical demands of football to be studied in detail (Bangsbo, 2014; Bangsbo 1994a; Bangsbo et al., 2006). Although players cover the majority of the distance (10-13km) by walking and low-intensity running, it is the high-intensity exercise activities which are associated with critical moments during a football match (Gregson et al., 2010). For example, a straight sprint is the most frequently observed activity prior to a goal being scored/conceded during match-play (Faude et al., 2012).
Sprint activity is supported by anaerobic energy provision. In a single 6-s sprint, glycogen will contribute approximately 50% to ATP turnover within the muscle (Cheetham et al., 1986). Thus, the consequence of repeated sprint activity is a net reduction in muscle glycogen concentrations (Saltin, 1973; Nevill et al., 1993) (Figure 1). Both type I and type II muscle fibre types have been reported to exhibit significant glycogen depletion, with approximately 80% of fibres being empty or almost empty ( i.e., lower than 200 mmol/kg d.w) of glycogen after a competitive game (Bendiksen et al., 2012). Although glycogen is depleted in both muscle fibre types, it may be the specific depletion of glycogen in type II muscle fibres that may result in the significant loss in power output during repetitive sprints (Greenhaff et al., 1994). Muscle glycogen concentrations of lower than approximately 200 mmol/kg d.w have been shown to significantly decrease the glycolytic rate (Bangsbo, 1994b). Furthermore, the depletion muscle glycogen in sub-cellular glycogen compartments, i.e., sarcoplasmic reticulum, results in associated reductions in muscle calcium (Ca2+ ) handling (Nielsen et al., 2011). If low glycogen influences the flux of calcium, the contractile property of the muscle will be compromised. Therefore, providing carbohydrate before and during exercise has a crucial role in maintaining an energy supply for these sub-cellular compartments. However, it is important to note that implications of depleted muscle glycogen go beyond that of energy provision for muscle contraction. Low muscle glycogen has numerous implications both for the innovation of muscle and the central nervous system (Nybo, 2003; Jensen & Richter 2012; Gejl et al., 2014). Thus, low muscle glycogen may result in the loss of skill execution, impair decision making and may also increase the risk of injury toward the end of a match (Medina et al., 2014; Rahnama et al., 2002). Interestingly, the overall distance achieved at high-speed has been identified as a distinguishing factor between top class and moderate to low level players (Mohr et al., 2003) (Figure 2). Furthermore, the overall success of a team is associated with smaller decrements in high intensity running toward the end of a match in comparison to less successful teams (Mohr et al., 2003). To this end, providing carbohydrate prior to football activity and the provision of carbohydrate during exercise are potent strategies which have been found to delay fatigue and improve performance.
Pre-match carbohydrate intake
The daily intake of carbohydrate should be proportionate to estimated fuel cost of the training session or match. It is unlikely that players complete matches or high-intensity sessions on a daily basis, especially during the season. Thus, for low-intensity, recovery or skill-based training players are advised to ingest 3-5 g of carbohydrate/ kg BM/ day. Whereas when players complete moderate training, approximately 1 h a day, carbohydrate intake of 5-7 g/kg BM/ day is recommended (Burke et al., 2011). Strategies to increase endogenous glycogen prior to competition have historically involved a “classical” 7 day model involving an initial “depletion” phase followed by a “loading phase” (Sherman, 1983). However, it is now known that the muscle of well-trained athletes is able to “supercompensate” glycogen stores without a prior “depletion” phase. Trained muscle also appears to have the ability to store more glycogen in comparison to untrained muscle and thus be more susceptible to “supercompensation” strategies (McInerney et al., 2005). Thus, a high carbohydrate diet providing 10 g of carbohydrate /kg BM/ day combined with appropriate rest can result in “supercompensated” muscle glycogen in as little as a 24-36 h (Bussau et al., 2002).
The importance of glycogen to football performance has resulted in the widely utilised “pre-match meal” strategy. The focus of the pre-match meal is to ingest an easy-to-digest high-carbohydrate meal 3-4 hours before exercise, to increase resting levels of glycogen in the muscle and liver. On match day the relative gains in endogenous glycogen stores achieved with carbohydrate feedings will be dependent upon starting concentrations and the training status of the muscle. However, as a guide, after an overnight fast, ingesting a meal containing 2.5 g of carbohydrate kg/ BM has been reported to increase muscle glycogen by 11-15% and liver glycogen by 33%, 3 h after ingestion (Taylor et al., 1996; Wu & Williams, 2006).
Immediately prior to the warm-up or match (depending on individual preference) players may ingest carbohydrate (25-30 g) to blunt the release of glucose from the liver, thus sparing the hepatic store of glycogen (Howlett et al., 1998). The role of liver glycogen is the regulation of blood glucose concentrations (euglycaemia: 4.0-5.5 mmol/l). At the onset of a match, muscular contraction will cause an increased uptake of glucose from the blood. In concert, liver glycogenolysis will be activated by the actions of glucagon and adrenaline. Interestingly, blood glucose has been reported to be elevated during repeated sprint activity and is rarely observed to decrease to concentrations that may impact performance (Krustrup et al., 2006). These findings would suggest that rate of glucose release from the liver is sufficient to compensate for the use of blood glucose throughout 90 min of football activity in well fed players. In fact, during football, blood glucose is only lowered during the halftime period. This is most likely a consequence of the continued uptake of glucose by the previously active muscle and a reduction in liver glycogenolysis, via a lowered catecholamine level during this period of recovery (Krustrup et al., 2006).
It is important to note that during prolonged match play, i.e., into extra time and penalties, blood glucose concentrations will fall and, if not replenished, may result in hypoglycaemia (Foskett et al., 2008). Symptoms of hypoglycaemia include sub-optimal functioning of the central nervous system which has obvious implication for football performance (Nybo, 2003). Conversely, increased blood glucose concentrations have been associated with superior “skill” performance in technical sports such as tennis (Vergauwen et al., 1998; McRae & Galloway, 2012). Thus, elevated blood glucose appears preferential when executing complex skills that require high levels of central nervous system activation, particularly during high-intensity intermittent activity (McMorris & Graydon, 1997; Winnick et al., 2005). Therefore, it is reasonable to conclude that maintaining or increasing blood glucose would improve “skill execution,” especially under circumstances of fatigue and/or hypoglycemia.
The ingestion of sufficient quantities of carbohydrate prior to a match is likely the most important strategy for football performance. This is because the opportunity to consume carbohydrate during the game is limited to infrequent breaks in play and the halftime period. To this end, there are several practical implications players and support staff should consider when adopting the pre-match meal strategies. First, the pre-match recommendation was originally based on the observation that liver glycogen stores are reduced to very low concentrations following an overnight fast. However, in the professional game, few matches begin before midday and many matches are now played in the evening. Under these circumstances, players have ample opportunity to replenish liver glycogen stores and top up muscle glycogen during the day. Thus, the timing of the pre-match meal should be changed to suit the match time and in consideration of other typical meals ingested during the day. Second, it is important to note the pre-match meal is typically a squad activity. Although all players must prepare as if they were to play, obviously this is not always the case. Club nutritionists and coaches should be aware to monitor the energy intake of players who consume a pre-match meal but do not play and modify their energy expenditure accordingly.
Carbohydrate during training and matches
Studies have been conducted showing a close association with carbohydrate ingestion and player performance during “live” matches. For example, in a study by Kirkendall et al., (1988) the physical performances of 10 players were captured on video on two separate occasions, separated by one day. For each match, players drank either a carbohydrate solution or sweetened placebo before the game and the same volume at halftime. It was reported that the players who drank the carbohydrate solution ran approximately 40% greater distance during the second half of the game, in comparison to when the placebo beverage was consumed (Kirkendall et al., 1988). Interestingly, a similar study in which players consumed 0.5 L of a 7% glucose solution 10 min before a practice match and the same volume again at halftime, reported a 39% reduction in muscle glycogen use compared to players drinking a sweetened placebo (Leatt & Jacobs, 1989). An important consideration when interpreting “performance” data during football matches is the high variability observed between games. For example, different tactical formations and levels of competition will have a great influence on the distance a player covers at high speed and sprint distances achieved (Gregson et al., 2010). Thus, although an interesting measure, assessing the impact that nutrition strategies have on match “performance” is challenging due to the complex interaction between physical and technical components. To this end, controlled experimental trials have offered excellent insights into the impact that carbohydrate ingestion during exercise has on multiple sprint performances and repeated skill execution.
Using a test specially designed to mimic the physical demands of football (Loughborough Intermittent Shuttle Run Test: LIST), Nicholas et al., (1995) performed a series of studies to investigate the effect that drinking a carbohydrate-electrolyte solution has on performance (Nicholas et al., 2000). In the first study, players drank a 6.9 % carbohydrate-electrolyte solution or sweetened placebo immediately before (5 ml/kg BM) exercise and during the 3-min breaks (2 ml/kg BM) between each 15-min block of exercise. This regime provided carbohydrate at a rate of approximately 1 g/min or 60 g/h. Repeated sprint performance was no different between trials. However, similar to observations from field studies, players were able to sustain high-intensity running for 2 min 10 s longer during the second part of the test when drinking the carbohydrate- electrolyte solution compared to the placebo (Nicholas et al., 1995). In a follow-up study, players repeated six 15-min blocks of intermittent running up to 90 min, drinking the same volume and concentration (6.9 %) of carbohydrate-electrolyte solution or sweetened placebo. In this study, muscle biopsy analysis revealed a significant reduction in muscle glycogen concentrations in both muscle fibre types. However, muscle glycogen use was reduced by 22% when players ingested the carbohydrate solution throughout exercise in comparison to placebo (Nicholas et al., 1994). Thus, the preservation of muscle glycogen offers a viable mechanism to explain why players are able to offset fatigue and sustain high-intensity running in the second half of football matches.
It is important to note the vital role of skill execution in football performance. Using a modified version of the LIST, McGregor et al., (1999) reported fluid ingestion during exercise had a significant benefit to the maintenance of soccer-specific skill (dribble track test) compared to no fluid intake. However, of relevance to this review, the ingestion of carbohydrate in addition to fluid has been reported to be superior to maintaining skill in comparison to the ingestion of fluid alone. Specifically, Ali and Williams (2009) developed a soccer passing and shooting performance test, which was performed before and immediately after the LIST (90 min). In this study, 16 male soccer players ingested either a 6.4% carbohydrate-electrolyte solution or placebo solution, 5 ml/kg BM before and 2 ml/kg BM every 15 min during exercise (~60 g of carbohydrate per hour). Passing performance was well maintained in both trials. However, a reduction in the deterioration of shooting performance was associated with carbohydrate ingestion (Ali & Williams, 2009). At a similar time Currell and colleagues also developed a football test protocol, which allowed the assessment of football specific skill performance (Currell et al., 2009). Importantly, the execution of football skills was reported to show little day-to-day variation, with CVs of 1.2%, 2.2% and 2.8% for agility, dribbling and kicking accuracy, respectively. In this study the ingestion of a 7.5% maltodextrin solution, 30 min before (6 ml/kg BM), at half-time (4 ml/kg BM) and routinely throughout exercise (1 ml/kg BM/ 12 min) was associated with a significant reduction in the deterioration of skill performance over 90 min of exercise, in comparison to the ingestion of a placebo solution (Figure 3). The ability to sustain skill execution during a football match has clear performance implications. For example, in Italy, teams with the smallest decrease in skill performance over a match were found to finish the season in a higher league position (Rampinini et al., 2009).
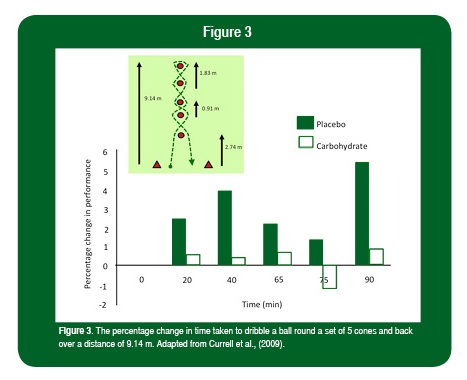
Interestingly, carbohydrate ingestion has been reported to improve both sprint (15 m) and skill performance during the early stages (0-45 min) of simulated football protocols (Ali & Williams, 2009; Currell et al., 2009). When adequate recovery time is allowed for phosphocreatine replenishment and sufficient glycogen remains in the muscle, a clear metabolic benefit of carbohydrate ingestion to support football performance is not immediately apparent. However, there is accumulating evidence that carbohydrate ingestion may have a “non-metabolic” central effect. Studies in running and cycling have reported a benefit on endurance performance by simply mouth rinsing and expectorating a carbohydrate solution (Rollo & Williams, 2011). The ergogenic effect may be mediated via the activation of brain pathways associated with reward and motivation, in response to carbohydrate recognition in the mouth. Nevertheless, to date, the benefits of mouth rinsing with a carbohydrate solution do not appear to extend to repeated sprint performance (Dorling & Earnest, 2013). Nevertheless, carbohydrate ingestion has also been reported to preserve the function of the central nervous system during prolonged exercise, which may have important implications for the successful execution of skills and decision making in football (Nybo, 2003).
The available literature suggests that the ingestion of an appropriate carbohydrate-electrolyte solution during football-specific exercise will benefit performance. Players with compromised glycogen stores will be able to sustain their level of skill proficiency and repeated sprint performance, in comparison to no fluid or the ingestion of fluid alone. As discussed, fatigue during football and prolonged exercise is associated with the decrease in muscle glycogen. The ingestion of carbohydrate has been shown to attenuate the decline in blood glucose concentrations during prolonged exercise and to spare muscle glycogen during a football match (Coyle et al., 1986; Leatt & Jacobs 1989). Thus, carbohydrate ingestion is advised for matches, where players aim to gain performance advantages and for intense training, where players should aim to gain maximal benefits from the session. The opportunity to ingest carbohydrate during football matches is often limited to unscheduled stoppages in play. It is vital that carbohydrate is readily available and players are aware of the benefits of ingesting a carbohydrate so that these opportunities are not missed. Finally, it is important to note that carbohydrate can be provided to players in a variety of different forms. For example, carbohydrate is oxidised effectively, whether it is provided in solid, i.e., in bars, chews, semi-solid gels, or in a drink (Pfeiffer et al., 2010). Thus, strategies to provide approximately 60 g of carbohydrate per hour can be modified according to player preference, however in context of other nutritional requirements such as the fluid needs of the player (Laitano et al., 2014). The main benefit of drinking well formulated carbohydrate-electrolyte beverages is that fuel and fluid needs are addressed simultaneously.
Recovery
Appropriate strategies to support a player’s recovery from training and matches are fundamental to the team’s overall ability to repeatedly perform. The aggressiveness of the recovery strategy will depend on when the player is next required to play, be it in training or competition and becomes increasingly important in tournament situations.
Interestingly, a complete depletion of muscle glycogen has been reported in a proportion of both slow and fast twitch muscle fibre types immediately after a football game (Krustrup et al., 2006). Of relevance to the recovery strategy is that the resynthesis of glycogen in these fibres is most rapid in the hours post exercise, in comparison to when carbohydrate is provided several hours later (Piehl 1974). A general recommendation to achieve high rates of glycogen resynthesis is for players to ingest approximately 1 g of carbohydrate /kg BM immediately after exercise (Ivy et al., 1988). Nicholas et al., (1997) completed a study to provide an example of how such a strategy impacts football performance. In this study, players performed the LIST to exhaustion on four occasions separated by one week. On one occasion players ingested a diet rich in carbohydrate and repeated the shuttle-run protocol 22 h later. The high-carbohydrate recovery diet resulted in an increase in the players’ normal daily energy intake from 2,600 kcal to 3,818 kcal. Absolute carbohydrate intake was increased from a daily average of 381 g to 705 g for the recovery period (5-10 g of carbohydrate/kg BM respectively). On the other occasion the players performed the shuttle-run protocol again. However, players ingested a mixed diet during the 22 h recovery period. The mixed diet contained their normal amounts of carbohydrate (381 g), with protein and fat consumed to match the energy intake of the carbohydrate diet. When players ingested the mixed diet, they could not achieve the previous day’s performance. However, the ingestion of the carbohydrate-rich diet was associated with an improved performance. Players were able to sustain high-intensity running for 3.3 min longer than that achieved on the previous day, which equated to an additional 7.4 min of running in comparison to the time achieved following the mixed diet (Nicholas et al., 1997).
Interestingly, more recent studies have suggested that the rate of muscle glycogen resynthesis may be slowed following a competitive football match. Glycogen stores were reported to be lower than pre-match concentrations 48 h post match, despite the ingestion of a high carbohydrate diet (Bangsbo et al., 2006; Krustrup et al., 2011). Football-specific activities, such as frequent change in direction and decelerations from sprints, have a high eccentric component. Eccentric contractions in combination with contact between players results in muscle damage, which in turn may impair glycogen synthesis (Krustrup et al., 2011). This phenomenon is not alleviated by a diet high in carbohydrate and whey protein (Gunnarsson et al., 2013). Of note, no super compensation of muscle glycogen concentrations have been reported 48 h after a football match, a typical response reported following prolonged running or cycling exercise.
Relevant to muscle damage, another interesting study regarding glycogen resynthesis and recovery was performed by Gregson et al., (2013). In an attempt to speed player recovery and reduce muscle soreness, cryotherapy “ice baths” have become a common strategy adopted by many football clubs. However, due to the vasoconstrictive consequence of cryotherapy, concerns have risen as to whether glycogen resynthesis would be impaired due to reduced availability of substrate to the muscle. Reassuringly, 10 min of lower limb cold water immersion (8°C), following exhaustive exercise, resulted in no impairment in glycogen restoration, in comparison to being seated at rest, when appropriate quantities of carbohydrate were ingested (Gregson et al., 2013).
It is important to note that the provision of protein should not be forgotten in context of an optimal recovery strategy post football match. In context of glycogen resynthesis, the co-ingestion of relatively small amounts of protein with carbohydrate can be used to augment postprandial insulin secretion and accelerate muscle glycogen synthesis rates (van Loon, 2007). Although, additional protein ingestion will not increase muscle glycogen resynthesis when sufficient quantities of carbohydrate are available (Betts & Williams, 2010). Nevertheless, research suggests that the inclusion of protein in concert with an appropriate carbohydrate intake will aid the rebuilding of muscle tissue and support adaptation specific to football performance (Res, 2014).
Finally, studies have reported that athletes’ “mood” state is better maintained by an increased daily intake of carbohydrate during intensive periods of training (5.5 g to 8.5 g of carbohydrate/ kg BM/ day) (Achten et al., 2004). During times of the season when players are exposed to more frequent matches, i.e., playing two games a week, the importance of maintaining the players’ vigour and motivation to train should not be underestimated.
Summary
Repeated sprint activity, characteristic of football, results in a net reduction in muscle glycogen concentrations. Low muscle glycogen concentrations have been associated with impaired performance, as measured by distance covered at high-intensity toward the latter stages of a match. The daily intake of carbohydrate should be proportionate to estimated fuel cost of the training session or match. On match days the ingestion of 2.5 g of carbohydrate/ kg BM, 3 h prior to exercise will top up stores of glycogen in the muscle and liver. Ingestion of 60 g of carbohydrate/ h, prior to and during (including half-time) exercise is associated with the maintenance of high-intensity running and skill execution. The preservation of both these factors, especially in the final stages of a game, has been identified as key performance attributes of top level football players and teams. Evidence is accumulating that carbohydrate ingestion also has a benefit on skill performance, however future studies are required to determine the exact mechanisms involved. Finally, it is important to note that this review has discussed the macronutrient carbohydrate, rather than food equivalents. Thus, the essential contribution of the sports nutritionist or sports dietician remains to translate the discussed research into practical meals based on the individual player’s preferences and needs.
References
Achten, J., S. L. Halson, L. Moseley, M. P. Rayson, A. Casey and A. E. Jeukendrup (2004). Higher dietary carbohydrate content during intensified running training results in better maintenance of performance and mood state. J Appl Physiol 96(4): 1331-1340.
Ali, A. and C. Williams (2009). Carbohydrate ingestion and soccer skill performance during prolonged intermittent exercise. J Sports Sci: 1-10.
Bangsbo, J. (1994a). Energy demands in competitive soccer. J Sports Sci 12 Spec No: S5-12.
Bangsbo, J. (1994b). The physiology of soccer--with special reference to intense intermittent exercise. Acta Physiol Scand Suppl 619: 1-155.
Bangsbo, J., M. Mohr and P. Krustrup (2006). Physical and metabolic demands of training and match-play in the elite football player. J Sports Sci 24(7): 665-674.
Bangsbo. J. Physiological Demands of Football. (2014) Sports Science Exchange. Vol. 27, No. 125, 1-6.
Bendiksen, M., R. Bischoff, M. B. Randers, M. Mohr, I. Rollo, C. Suetta, J. Bangsbo and P. Krustrup (2012). The Copenhagen Soccer Test: physiological response and fatigue development. Med Sci Sports Exerc 44(8): 1595-1603.
Betts, J. A. and C. Williams (2010). Short-term recovery from prolonged exercise: exploring the potential for protein ingestion to accentuate the benefits of carbohydrate supplements. Sports Med 40(11): 941-959.
Burke, L. M., J. A. Hawley, S. H. Wong and A. E. Jeukendrup (2011). Carbohydrates for training and competition. J Sports Sci 29 Suppl 1: S17-27.
Bussau, V. A., T. J. Fairchild, A. Rao, P. Steele and P. A. Fournier (2002). Carbohydrate loading in human muscle: an improved 1 day protocol. Eur J Appl Physiol 87(3): 290-295.
Cheetham, M. E., L. Boobis, S. Brooks and C. Williams (1986). Human muscle metabolism during sprint running in man. Journal of Applied Physiology 61: 54-60.
Coyle, E. F., A. R. Coggan, M. K. Hemmert and J. L. Ivy (1986). Muscle glycogen utilization during prolonged strenuous exercise when fed carbohydrate. Journal of Applied Physiology 61(1): 165-172.
Currell, K., S. Conway and A. E. Jeukendrup (2009). Carbohydrate ingestion improves performance of a new reliable test of soccer performance. Int J Sport Nutr Exerc Metab 19(1): 34-46.
Dorling, J. L. and C. P. Earnest (2013). Effect of carbohydrate mouth rinsing on multiple sprint performance. J Int Soc Sports Nutr 10(1): 41.
Faude, O., T. Koch and T. Meyer (2012). Straight sprinting is the most frequent action in goal situations in professional football. J Sports Sci 30(7): 625-631.
Foskett, A., C. Williams, L. Boobis and K. Tsintzas (2008). Carbohydrate availability and muscle energy metabolism during intermittent running. Med Sci Sports Exerc 40(1): 96-103.
Gejl, K. D., L. G. Hvid, U. Frandsen, K. Jensen, K. Sahlin and N. Ortenblad (2014). Muscle Glycogen Content Modifies SR Ca2+ Release Rate in Elite Endurance Athletes. Med Sci Sports Exerc 46(3): 496-505.
Greenhaff, P. L., M. E. Nevill, K. Soderlund, K. Bodin, L. H. Boobis, C. Williams and E. Hultman (1994). The metabolic responses of human type I and II muscle fibres during maximal treadmill sprinting. J Physiol 478 ( Pt 1): 149-155.
Gregson, W., R. Allan, S. Holden, P. Phibbs, D. Doran, I. Campbell, S. Waldron, C. H. Joo and J. P. Morton (2013). Postexercise cold-water immersion does not attenuate muscle glycogen resynthesis. Med Sci Sports Exerc 45(6): 1174-1181.
Gregson, W., B. Drust, G. Atkinson and V. Di Salvo (2010). Match-to-match variability of high speed activities in premier league soccer. International Journal of Sports Medicine 31(4): 237-242.
Gunnarsson, T. P., M. Bendiksen, R. Bischoff, P. M. Christensen, B. Lesivig, K. Madsen, F. Stephens, P. Greenhaff, P. Krustrup and J. Bangsbo (2013). Effect of whey protein- and carbohydrate-enriched diet on glycogen resynthesis during the first 48 h after a soccer game. Scand J Med Sci Sports 23(4): 508-515.
Howlett, K., D. Angus, J. Proietto and M. Hargreaves (1998). Effect of increased blood glucose availability on glucose kinetics during exercise. J Appl Physiol (1985) 84(4): 1413-1417.
Ivy, J. L., A. L. Katz, C. L. Cutler, W. M. Sherman and E. F. Coyle (1988). Muscle glycogen synthesis after exercise: effect of time of carbohydrate ingestion. Journal of Applied Physiology 64(4): 1480-1485.
Jensen, T. E. and E. A. Richter (2012). Regulation of glucose and glycogen metabolism during and after exercise. J Physiol 590(Pt 5): 1069-1076.
Jeukendrup, A. E. (2003). Modulation of carbohydrate and fat utilization by diet, exercise and environment. Biochem Soc Trans 31(Pt 6): 1270-1273.
Kirkendall, D., C. Foster, J. Dean, J. Grogan and N. Thompson (1988). Effect of glucose polymer supplementation on performance of soccer players. In: T. Reilly, A. Lees, K. Davids, and W. Murphy, eds. Science and Football.(London: E & FN Spon.): 33-41.
Krustrup, P., M. Mohr, A. Steensberg, J. Bencke, M. Kjaer and J. Bangsbo (2006). Muscle and blood metabolites during a soccer game: implications for sprint performance. Med Sci Sports Exerc 38(6): 1165-1174.
Krustrup, P., N. Ortenblad, J. Nielsen, L. Nybo, T. P. Gunnarsson, F. M. Iaia, K. Madsen, F. Stephens, P. Greenhaff and J. Bangsbo (2011). Maximal voluntary contraction force, SR function and glycogen resynthesis during the first 72 h after a high-level competitive soccer game. Eur J Appl Physiol 111(12): 2987-2995.
Laitano. O. Runco. J.L and L. Baker. Hydration Science and Strategies in Football. (2014) Sports Science Exchange. Vol. 27, No. 128, 1-7.
Leatt, P. B. and I. Jacobs (1989). Effect of glucose polymer ingestion on glycogen depletion during a soccer match. Can J Sport Sci 14(2): 112-116.
McInerney, P., S. J. Lessard, L. M. Burke, V. G. Coffey, S. L. Lo Giudice, R. J. Southgate and J. A. Hawley (2005). Failure to repeatedly supercompensate muscle glycogen stores in highly trained men. Med Sci Sports Exerc 37(3): 404-411.
McGregor, S. J., C. Nicholas, W., H. W. Lakomy and C. Williams (1999). The influence of intermittent high-intensity shuttle running and fluid ingestion on the performance of a football skill. . Journal of Sports Sciences 17(11): 895-903.
McMorris, T. and J. Graydon (1997). The effect of exercise on cognitive performance in soccer-specific tests. J Sports Sci 15(5): 459-468.
McRae, K. A. and S. D. Galloway (2012). Carbohydrate-electrolyte drink ingestion and skill performance during and after 2 hr of indoor tennis match play. Int J Sport Nutr Exerc Metab 22(1): 38-46.
Medina. D, Lizarraga. A and F. Drobnick. Injury Prevention and Nutrition in Football. (2014) Sports Science Exchange. Vol. 27, No. 132, 1-5.
Mohr, M., P. Krustrup and J. Bangsbo (2003). Match performance of high-standard soccer players with special reference to development of fatigue. J Sports Sci 21(7): 519-528.
Nevill, M. E., C. Williams, D. Roper, C. Slater and A. M. Nevill (1993). Effect of diet on performance during recovery from intermittent sprint exercise. J Sports Sci 11(2): 119-126.
Nicholas, C., C. Williams, L. Boobis and N. Little (1994 ). Effect of ingesting a carbohydrate-electrolyte beverage on muscle glycogen utilization during high intensity, intermittent shuttle running. Clinical Science 87 (suppl: 26-27.).
Nicholas, C. W., P. A. Green, R. D. Hawkins and C. Williams (1997). Carbohydrate intake and recovery of intermittent running capacity. Int J Sport Nutr 7(4): 251-260.
Nicholas, C. W., F. E. Nuttall and C. Williams (2000). The Loughborough Intermittent Shuttle Test: a field test that simulates the activity pattern of soccer. J Sports Sci 18(2): 97-104.
Nicholas, C. W., C. Williams, H. K. Lakomy, G. Phillips and A. Nowitz (1995). Influence of ingesting a carbohydrate-electrolyte solution on endurance capacity during intermittent, high-intensity shuttle running. J Sports Sci 13(4): 283-290.
Nielsen, J., H. C. Holmberg, H. D. Schroder, B. Saltin and N. Ortenblad (2011). Human skeletal muscle glycogen utilization in exhaustive exercise: role of subcellular localization and fibre type. J Physiol 589(Pt 11): 2871-2885.
Nybo, L. (2003). CNS fatigue and prolonged exercise: effect of glucose supplementation. Med Sci Sports Exerc 35(4): 589-594.
Pfeiffer, B., T. Stellingwerff, E. Zaltas and A. E. Jeukendrup (2010). Oxidation of solid versus liquid CHO sources during exercise. Med Sci Sports Exerc 42(11): 2030-2037.
Piehl, K. (1974). Time course for refilling of glycogen stores in human muscle fibres following exercise-induced glycogen depletion. Acta Physiol Scand 90(2): 297-302.
Rahnama, N., T. Reilly and A. Lees (2002). Injury risk associated with playing actions during competitive soccer. Br J Sports Med 36(5): 354-359.
Rampinini, E., F. M. Impellizzeri, C. Castagna, A. J. Coutts and U. Wisloff (2009). Technical performance during soccer matches of the Italian Serie A league: effect of fatigue and competitive level. J Sci Med Sport 12(1): 227-233.
Res, P. Recovery Nutrition for Football Players. (2014) Sports Science Exchange. Vol. 27, No. 129, 1-5.
Rollo, I. and C. Williams (2011). Effect of mouth-rinsing carbohydrate solutions on endurance performance. Sports Med 41(6): 449-461.
Saltin, B. (1973). Metabolic fundamentals in exercise. Medicine and Science in Sports and Exercise 5(3): 137-146.
Sherman, W. (1983). Carbohydrates, muscle glycogen, and muscle glycogen supercompensation. Ergogenic aids in sports. M. H. Williams. Champaign, IL., Human, Kinetics Publishers.: 1-25.
Taylor, R., I. Magnusson, D. L. Rothman, G. W. Cline, A. Caumo, C. Cobelli and G. I. Shulman (1996). Direct assessment of liver glycogen storage by 13C nuclear magnetic resonance spectroscopy and regulation of glucose homeostasis after a mixed meal in normal subjects. The Journal of Clinical Investigation 97(1): 126-132.
van Loon, L. J. (2007). Application of protein or protein hydrolysates to improve postexercise recovery. Int J Sport Nutr Exerc Metab 17 Suppl: S104-117.
Vergauwen, L., F. Brouns and P. Hespel (1998). Carbohydrate supplementation improves stroke performance in tennis. Med Sci Sports Exerc 30(8): 1289-1295.
Winnick, J. J., J. M. Davis, R. S. Welsh, M. D. Carmichael, E. A. Murphy and J. A. Blackmon (2005). Carbohydrate feedings during team sport exercise preserve physical and CNS function. Med Sci Sports Exerc 37(2): 306-315.
Wu, C. L. and C. Williams (2006). A low glycemic index meal before exercise improves endurance running capacity in men. Int J Sport Nutr Exerc Metab 16(5): 510-527.