Sports Science Exchange 88
VOLUME 16 (2003) NUMBER 1
HYPONATREMIA IN ATHLETES
Bob Murray, Ph.D.
Director
Gatorade Sports Science Institute
Barrington, Illinois |
E. Randy Eichner, M.D.
Professor of Medicine
Team Internist, Oklahoma Sooners
Department of Medicine
University of Oklahoma Medical Center
Oklahoma City, Oklahoma |
John Stofan, M.S.
Senior Research Scientist
Gatorade Sports Science Institute
Barrington, Illinois |
|
KEY POINTS
- Fatal hyponatremia in athletes is rare, but it has claimed the lives of marathon runners and military recruits. For that reason, sports health professionals should understand the causes of hyponatremia and the practical steps that can be taken to reduce the risk.
- Hyponatremia occurs when blood sodium concentration falls to an abnormally low level, prompting a rapid and dangerous swelling of the brain that can result in seizures, coma, and death.
- Although hyponatremia is often associated with prolonged exercise, it can also occur at rest when too much fluid is ingested too quickly.
- Excessive drinking is a key risk factor for hyponatremia, but it is possible for hyponatremia to occur—without excessive drinking—in dehydrated athletes during very prolonged exercise as a result of large sodium losses in sweat.
- The risk of hyponatremia can be reduced by making certain that fluid intake does not exceed sweat loss and by ingesting sodium containing beverages or foods to help replace the sodium lost in sweat.
- For most athletes, dehydration remains the primary challenge to physiological homeostasis and performance, but hyponatremia should be recognized as a possible threat to those athletes who drink more fluid than they lose in sweat.
INTRODUCTION
On Sunday, December 2, 2001, in the comic section of the Chicago Tribune, the Peanuts comic strip dealt with hyponatremia. Team doctor Snoopy trotted out onto the field and deduced that Woodstock was suffering from hyponatremia. Snoopy correctly determined that this electrolyte imbalance could be corrected by the right combination of water and salt. Woodstock was allowed to drink the water, but Snoopy sprinkled the salt on his feathers. In this case, comic license overruled good medical practice, although Woodstock evidently survived Snoopy's inappropriate treatment. That such a rare disorder was featured in a popular comic strip attests to a growing interest in the topic among the general public as well as among sports health professionals.
That hyponatremia can prove fatal to otherwise healthy athletes is reason enough for sports health professionals to be aware of what the risk factors are and how the disorder can be prevented. Although the incidence of fatal hyponatremia is rare, case reports and descriptive data suggest that non-fatal hyponatremia may be common. Estimates of the frequency of hyponatremia associated with prolonged exercise (e.g., marathons and Ironman-distance triathlons) span a wide range, in some cases exceeding 30% of the athletes tested (O'Toole et al., 1995). However, data from the US military indicate an incidence of hyponatremia of only about 0.10 per 1,000 soldier-years (Craig, 1999), far below the rates reported in athletes (Davis et al. 1999; O'Toole et al., 1995; Speedy et al., 1999). The wide disparity in incidence rates may partly reflect differences in case finding and severity. For example, studies on athletes often use a subset of race entrants, those seen in the medical tent. Some of these athletes have only mild hyponatremia and no clear-cut symptoms from it. Incidence statistics from the military and some studies on athletes key on hospitalized cases, so mild cases of hyponatremia could be missed. We need to study larger cohorts of athletes to better characterize the risk of hyponatremia. Still, the current data warrant that medical personnel consider hyponatremia as a possible cause of collapse during or following prolonged exercise.
RESEARCH REVIEW
What is hyponatremia?
Hyponatremia is a disorder in fluid-electrolyte balance that results in an abnormally low plasma sodium concentration (<135 mmol/liter; normal = 136-142 mmol/liter). A sustained decrease in plasma sodium concentration disrupts the osmotic balance across the blood-brain barrier, resulting in a rapid influx of water into the brain. This causes brain swelling and a cascade of increasingly severe neurological responses (confusion, seizure, coma) that can culminate in death from rupture of the brainstem. The faster and lower the blood sodium falls, the greater the risk of life-threatening consequences. A decrease in plasma sodium concentration to 125-135 mmol/liter usually results in either no noticeable symptoms or in relatively modest gastrointestinal disturbances such as bloating or mild nausea. Below 125 mmol/liter, the symptoms become more severe and include throbbing headache, vomiting, wheezy breathing, swollen hands and feet, restlessness, unusual fatigue, confusion, and disorientation (Adrogué & Madias, 2000). When plasma sodium concentration drops below 120 mmol/liter, seizure, respiratory arrest, coma, permanent brain damage, and death become more likely. However, some athletes have survived hyponatremia of <115 mmol/liter (Backer et al., 1993), whereas others have died at >120 mmol/liter (Gardner, 2002a).
The hyponatremia that occurs in athletes is most often characterized by hypo-osmolality (hypotonicity) of plasma. This condition is known as hypotonic or dilutional hyponatremia, i.e., more water than normal for the amount of substances dissolved in the plasma. Hyponatremia can also occur with normal or even high plasma osmolality. Isotonic hyponatremia (normal relation between water and dissolved substances) is rare, but can result from retention of sodium-free isotonic fluids in the extracellular fluid compartment, an event that is relevant to some hospital procedures (e.g., isotonic mannitol infusions) but not to sports. Hypertonic hyponatremia (less water than normal for the amount of substances dissolved in plasma) can occur with severe hyperglycemia or with glycerol loading (Freund et al., 1995) when water retained in the vascular space is sufficient to temporarily reduce blood sodium concentration.
Who is at risk of hyponatremia?
In general, athletes who drink too much before and during prolonged exercise in warm, humid climates are at risk of developing hyponatremia. Although larger athletes are not immune to hyponatremia, small, slow athletes who sweat a lot, excrete a salty sweat, and are overzealous in their drinking habits are theoretically at greater risk. A small body means it takes less fluid to dilute the extracellular fluid (ECF). Slow runners, triathletes, and cyclists have more time and easier opportunities to drink excessively.
Large sweat losses and/or salty sweat speed sodium loss. Athletes who are hypervigilant about hydration can accelerate dilution of the ECF, especially when they rely on water as their primary fluid. Athletes who start already hyponatremic from excessive drinking in the days or hours before the race are at particular risk of more-severe hyponatremia during the race because less fluid is required to drop plasma sodium to dangerous levels.
What causes hyponatremia in athletes?
The possible causes of exercise-related hyponatremia are many and varied. One hypothesis is SIADH, the syndrome of inappropriate antidiuretic hormone (ADH) response. When this occurs, there is reduced urine production and greater retention of ingested fluid in the presence of fluid overload. A second hypothesis is the sequestering of water in the gut (resulting in post-race dilution when the water is absorbed). Another is abuse of non-steroidal anti-inflammatory drugs (NSAIDs), which may alter kidney function and decrease urine production. Finally, hyponatremia may be caused by abnormally high sodium losses in sweat. Excessive drinking is often, but not always, the common denominator in these scenarios. However, even in the absence of other provocations, excessive drinking alone can result in hyponatremia, as has occurred in people who have ingested large volumes of fluid (e.g., 3 liters—more than 3 quarts—of water in an hour) trying to produce a dilute urine to escape detection of banned drugs during drug tests (Zehlinger et al., 1996; Gardner, 2002b).
In the simplest terms, hyponatremia results from some combination of abnormal water retention and/or sodium loss (see Figure 1). Water retention can occur from excessive water retention by the kidneys or from drinking too much water. In athletes, sodium losses in sweat exacerbate the problem. What might cause excessive water retention or intake? A review article by Adrogué and Madias (2000) contained over 60 possible causes by which impaired renal water excretion or excessive water intake can result in hyponatremia. However, most of these apply to hospitalized patients with other medical issues (e.g., cancer, bowel obstruction, acute psychosis, adrenal insufficiency, and inappropriate ADH secretion), not to healthy athletes.
Too much of a good thing?
While it is possible that hyponatremia in athletes can result from SIADH, the overuse of NSAIDs, or unusual electrolyte shifts, the more likely scenario is that the combination of excessive drinking and salt loss reduces plasma sodium concentration. In healthy athletes in a race, urine production normally decreases and sodium loss (via sweat) increases, setting the stage for hyponatremia if too much fluid is ingested or retained.
Under these circumstances, excessive drinking will unavoidably decrease the plasma sodium concentration. However, under most conditions, abnormally large volumes of fluid must be ingested to create hyponatremia, a risk in individuals who mistakenly assume that excessive drinking is not dangerous. For example, the nine cases of hyponatremia among U.S. Marine recruits that occurred on a single day in the summer of 1995 resulted from each soldier drinking 10 to 22 quarts of water over a few hours (Gardner, 2002a). Their plasma sodium concentrations ranged from 114—133 mmol/liter. Fortunately, all survived after emergency treatment. In another example, Davis et al. (2001) chronicled 26 cases of symptomatic hyponatremia from the 1998 and 1999 San Diego Marathons. The average finish time for the 26 runners was 5 hours, 38 minutes (range = 4:00 to 6:34), and many runners admitted drinking as much fluid as possible during and after the event. How much did they drink? That remains unclear, but plasma sodium values ranged from 117 to 134 mmol/liter, so excessive drinking is a strong possibility. Moreover, sweat sodium loss—although not measured in this study—likely contributed to the problem.
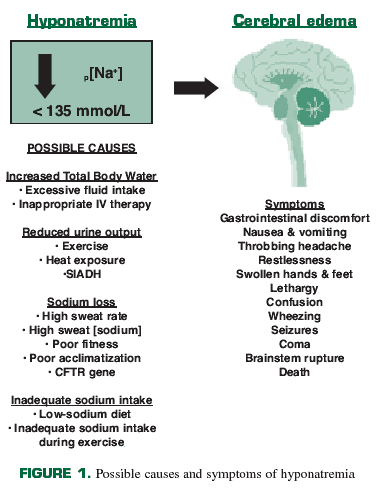
During exercise, and particularly during exercise in the heat, urine production declines 20—60% from resting values due to a decrease in kidney blood flow, which results in a decreased rate of urine production (Zambraski, 1990). At the same time, the kidneys are reabsorbing both sodium and water in response to sympathetic nerve stimuli and to exercise-induced increases in aldosterone (Zambraski, 1990). As a result, exercising humans have a reduced capacity to excrete water, a normal physiological response that nevertheless increases the risk that excessive drinking will lead to hyponatremia.
The capacity of the kidneys to process excess fluid can also be overwhelmed at rest. Whenever fluid intake exceeds the maximal rate of urine production, plasma sodium will unavoidably fall. Speedy et al. (2001) and Noakes et al. (2001) showed that plasma sodium levels can quickly plummet when resting subjects overdrink water. The volumes of water ingested in these studies (~1.5 liters/hour over 2-3 hours) could easily be consumed by an overzealous drinker either the evening before or the morning of a race. Most adults can drink 1.5 liters (1.6 quarts) or more per hour, exceeding maximal urine production of about 1,000 ml/hour (Zambraski, 1990). Under most circumstances, modest overdrinking presents little threat of hyponatremia. In fact, most people overdrink periodically throughout the day, with the excess water soon lost as urine. However, some athletes may drink large volumes of fluid in the days preceding the race in a misguided attempt to stay well hydrated or may inadvertently overdrink because their daily fluid intake remains high even though their training load has decreased. For example, Eichner (2002) noted that a woman who experienced hyponatremia in a marathon race drank 10 liters (10.6 quarts) of fluid the night before. Regardless of the reason, excessive drinking before, during, and after exercise dramatically raises the risk of hyponatremia.
Are females at greater risk of hyponatremia?
In a report on hyponatremia following the San Diego Marathon, 23 of the 26 cases of hyponatremia were females (Davis et al. 2001). Ayus et al. (2000) reported that five of seven hyponatremic runners in their study were female. Hyponatremia was three times more common in women than in men in a study of finishers at the 1997 New Zealand Ironman triathlon (Speedy, 1999). Backer et al. (1999) found that six of seven hikers who suffered from hyponatremia in the Grand Canyon were female. These findings led to the hypothesis that females are somehow more susceptible to hyponatremia (Eichner, 2002). This gender trend, however, may be more behavioral than biological. For example, the incidence of hyponatremia in the US military reflects the gender distribution in the military: 85% male, 15% female (Montain et al., 2001). In the 2000 Houston Marathon, the incidence of hyponatremia was similar in males and females (Hew et al., 2003). Anecdotes suggest that females are more vigilant drinkers (witness the propensity for women to carry bottled water with them throughout the day) and female athletes may be more likely to heed, and sometimes exceed, advice from coaches and experts. So women, more than men, may overdo the advice that "staying well hydrated is good for health and performance."
Even if the risk of hyponatremia is not greater in females, the clinical outcome for females is worse than for males (Ayus et al., 1992). This may be because estrogen inhibits the enzyme responsible for moving potassium out of brain cells (Arieff, 1986). The response to the swelling caused by hyponatremia is to transport potassium out of the cell, thereby reducing intracellular osmolality and offsetting the influx of more water into the cell (Adrogué & Madias, 2000). Accordingly, if the sodium-potassium ATPase enzyme is inhibited by estrogen, the clinical outcome of hyponatremia may be more grave. According to Ayus and Arieff (1992), young women, who have relatively high levels of estrogen, are 25 times more likely to die or have permanent brain damage as a result of postoperative hyponatremic brain swelling compared to men or postmenopausal women, who have relatively low levels of estrogen.
Why is salty sweat a risk factor?
Highly fit athletes who are well acclimated to exercise in warm environments usually excrete sweat with sodium concentrations less than 40 mmol/liter of sodium, because the capacity of the sweat glands for sodium conservation is enhanced with heat acclimation and improved aerobic fitness. This reduction in sodium loss not only helps protect blood volume, but also reduces the risk of hyponatremia. However, relatively unfit and unacclimated individuals, and even some highly trained athletes, may excrete sweat containing sodium concentrations greater than 60 mmol/liter. These "salty sweaters", particularly those who have high sweat rates, can lose large amounts of sodium. For example, during an Ironman-distance triathlon, an athlete with a normal sweat sodium concentration of 40 mmol/liter, losing a modest 1.0 liter of sweat each hour, would lose 11.0 grams of sodium (contained in 27.6 grams of sodium chloride) in 12 hours of racing. Of course, an athlete with saltier sweat would lose considerably more. The important consideration is that salt loss through sweating can be a contributing factor to the etiology of hyponatremia, with larger salt losses conferring greater risk.
In a review of exertional hyponatremia, Montain et al. (2001) provided estimates of the plasma sodium concentration changes that will occur during prolonged exercise when water intake is equal to sweat loss. Their calculations indicate that athletes who excrete sweat containing high levels of sodium are at greater risk of hyponatremia because it takes less water intake to induce dangerously low blood sodium levels. Using their calculations, it can also be estimated that high sweat sodium losses alone can result in hyponatremia during prolonged exercise (e.g., 9 hours or more) even in the absence of overdrinking. In addition, their calculations demonstrate that smaller athletes will be at a greater risk of hyponatremia because they have less ECF to dilute. (Smaller ECF volumes may be one reason why female athletes appear at greater risk of hyponatremia. For example, even if a male and female have the same body mass, the female has less total body water and less ECF, increasing the relative risk of hyponatremia.)
Hyponatremia is usually caused by a combination of sweat sodium loss and excessive water intake. As suggested by Hiller (1989), it is possible for dehydrated athletes to become hyponatremic during prolonged events if they lose a lot of sodium in sweat and drink water (and/or other salt-poor beverages) to replace most but not all of their sweat. For example, if in a long, hot race, an athlete loses 10 liters of salty sweat and drinks 8 liters of water, the athlete will become both dehydrated and hyponatremic. This is consistent with observations of physicians at the Hawaii Ironman triathlon, where some finishers arrive at the medical tent with signs and symptoms of dehydration (e.g., sunken eyes, tented skin—when skin on the back of the hand is pinched, it remains folded or "tented", ongoing low blood pressure when standing, etc.), yet are also hyponatremic. However, additional research is needed to confirm the likelihood of hyponatremia in dehydrated athletes.
Are some people genetically predisposed to hyponatremia due to high sweat sodium losses?
A distinguishing characteristic of cystic fibrosis (CF) is salty sweat. CF is caused by a defect in a gene that encodes for a protein involved in the transport of chloride—and indirectly sodium—out of the sweat duct. (Davis, 2001; Quinton, 1999). This would explain the predominant characteristic of CF—high levels of sodium and chloride in sweat. CF is most prevalent in those of Northern and Central European heritage, where up to 1 in 20 carries a recessive gene for CF. Less than 1% of CF patients have sweat sodium or chloride levels less than 60 mmol/liter (Davis, 2001); for that reason, high sweat electrolyte concentrations are used as diagnostic criteria. Although there is some evidence that individuals with CF are susceptible to hyponatremia (Montain et al., 2001; Smith et al., 1995), additional research is needed to determine just how prevalent the CF gene is among those who develop hyponatremia.
What fluid-replacement guidelines should athletes follow?
It is well established that dehydration resulting from sweat loss compromises important physiological functions and impairs performance. As a result, it is necessary to ingest fluid during exercise to reduce the risk of heat-related illness, maintain physiological functions, and improve performance (Murray, 2000). This advice is reflected in a number of position stands from a variety of professional organizations (see below). Because sweat loss varies widely among people, the amount of fluid required during exercise to prevent significant dehydration must also vary widely. For example, some people are very efficient sweaters and excrete less than a liter of sweat per hour, even under adverse environmental conditions. In others, sweat loss during physical activity can reach very high levels, at times exceeding two or even three or more liters per hour (Murray, 2000).
It has been suggested that athletes restrict fluid intake to no more than 400—800 ml per hour during exercise to reduce the risk of hyponatremia (Noakes, 2002). This is sound advice for those athletes who sweat at such low rates, but faulty advice for those who sweat substantially more. Those athletes can benefit from rates of fluid intake that more closely match their sweat losses, without increasing their risk of hyponatremia, provided that sodium is also ingested during exercise. Noakes (2002) argues that athletes have been told that they should "drink as much as possible during exercise," advice that might predispose some to excessive drinking and hyponatremia. There are several recent scientific position stands (see below) that provide guidelines for fluid replacement before, during, and following exercise, none of which suggest that athletes should "drink as much as possible during exercise." As would be expected, the language of the position stands is not uniform, but the recommendations are similar in both intent and content.
American College of Sports Medicine (1996): "It is recommended that individuals drink about 500 ml (about 17 ounces) of fluid about 2 h before exercise to promote adequate hydration and allow time for the excretion of excess ingested water. During exercise, athletes should start drinking early and at regular intervals in an attempt to consume fluids at a rate sufficient to replace all the water lost through sweating (i.e., body weight loss), or consume the maximal amount that can be tolerated."
American Academy of Pediatrics (2000): "Before prolonged physical activity, the child should be well hydrated. During the activity, periodic drinking should be enforced (e.g., each 20 minutes 150 ml [5 oz] of cold tap water or a flavored salted beverage for a child weighing 40 kg (88 lbs) and 250 ml [9 oz] for an adolescent weighing 60 kg (132 lbs)), even if the child does not feel thirsty. Weighing before and after a training session can verify hydration status if the child is wearing little to no clothing."
American Dietetics Association, Dietitians of Canada, and American College of Sports Medicine (2000): "Athletes should drink enough fluid to balance their fluid losses. Two hours before exercise, 400 to 600 ml (14 to 22 oz) of fluid should be consumed, and during exercise, 150 to 350 ml (6 to 12 oz) of fluid should be consumed every 15 to 20 minutes depending on tolerance."
National Athletic Training Association (2000): "To ensure proper pre-exercise hydration, the athletes should consume approximately 500 to 600 ml (17 to 20 oz) of water or a sports drink 2 to 3 hours before exercise and 200 to 300 ml (7 to 10 oz) of water or a sports drink 10 to 20 minutes before exercise. Fluid replacement should approximate sweat and urine losses and at least maintain hydration at less than 2% bodyweight reduction. This generally requires 200 to 300 ml (7 to 10 oz) every 10 to 20 minutes."
These guidelines recognize that drinking adequately during exercise improves performance and reduces the risk of heat-related illness, but none suggests that athletes "drink as much fluid as possible during exercise." The examples used in each paper to characterize fluid intake during exercise contain values for fluid consumption that accurately portray "average" sweat losses. Obviously, athletes who lose more than the average amount of sweat will require more than the average amount of fluid intake to prevent significant dehydration. Those athletes who sweat at lower-than-average rates should drink less. On those occasions when it is not possible to closely match fluid intake with sweat rate, the guidelines suggest that athletes ingest as much fluid as they can comfortably tolerate. This advice recognizes that drinking to minimize dehydration is a good thing, even when athletes can't drink enough to keep pace with large sweat losses. The various guidelines on fluid replacement during exercise are just that - guidelines to help athletes meet their individual needs.
This common-sense logic underscores the value of athletes recording their body weights before and after training sessions to determine if their fluid intakes match their sweat losses. For example, if a 130-lb (60-kg) distance runner weighs 5 lbs (2.3 kg) less after a 10-mile (16-km) run, this is a clear indication that fluid intake should have been greater. In contrast, a 260-lb (118-kg) football player who weighs only 2 lbs (0.9 kg) less after a tough practice has done a fairly good job of drinking during practice. And a female triathlete who weighs 1-lb (0.45 kg) more after a long training session on her bike should cut back on drinking during her next ride.
Does metabolic water production during exercise increase the risk of hyponatremia?
Noakes (2002) suggested that using changes in body weight during exercise as an indication of fluid needs might provide a distorted sense of an athlete's actual need for fluid. He argued that substrate metabolism and the breakdown of muscle and liver glycogen might contribute a substantial amount of water during prolonged exercise. If this were true, then the pre-to-post-exercise change in body weight would not give an accurate representation of fluid needs. According to this hypothesis, drinking to replace weight loss might increase the risk of hyponatremia. Fortunately, there is little likelihood that this is the case.
As would be expected, metabolic water production—a byproduct of the combustion of carbohydrate and fat—increases with exercise intensity because carbohydrate and fat oxidation also increase. While there is no doubt that fuel metabolism contributes some water during exercise, the total production is small (Pivarnik et al., 1984). For example, during treadmill running at 74%VO2max, metabolic water production averaged 2.4 grams/minute, or about 144 grams/hour. In comparison, sweat loss was 20.9 grams/minute or about 1,200 grams/hour (Pivarnik et al., 1984). Because only about 8% of body water is contained within the vascular space, only about 12 ml/hour would be contributed to the plasma volume by metabolic water production at 74%VO2max.
Can water "released" with glycogen breakdown contribute to hyponatremia during prolonged exercise? Each gram of glycogen in muscle and liver is supposedly associated with about 3-5 grams of water (Olsson & Saltin, 1970). Does that mean that the oxidation of 400 grams of glycogen during prolonged exercise releases 1.2 to 2.0 liters of water? No, it does not. First, no one is certain how much water is stored along with glycogen in muscle because there is no consistent relationship between increases in muscle glycogen content and muscle water content (Sherman et al., 1982). Second, whatever water is associated with glycogen storage is already part of the total body water. That is, the water contributes to fluid-electrolyte balance whether or not it is associated with glycogen. Third, when glycogen breaks down during exercise, most of the water molecules will remain in the intracellular space unless there is an osmotic gradient favoring their movement into the extracellular space. This is not likely to occur during exercise because the normal increase in intracellular osmolality retains water within the muscle. Finally, only about 8% of whatever water might leave the intracellular space will find its way into the plasma volume.
SUMMARY
There is little doubt that proper hydration benefits physiological function, performance, and health. There is also little doubt that excessive drinking can create a potentially life-threatening situation. It appears that excessive fluid intake is the primary cause in most cases of hyponatremia. For that reason, it is vital that we continue to teach athletes about proper hydration and about the potential danger of excessive drinking (See back cover of this article for practical advice for athletes and others.)
REFERENCES
Adrogué, H.J., and N.E. Madias (2000). Hyponatremia. New Engl. J. Med. 342:1581-1589.
American Academy of Pediatrics (2000). Climatic heat stress and the exercising child and adolescent. Pediatrics 106:158-159.
American Dietetic Association, Dietitians of Canada, and American College of Sports Medicine (2000). Nutrition and athletic performance. J. Amer. Diet. Assoc. 100:1543-1556.
American College of Sports Medicine (1996). Position stand on exercise and fluid replacement. Med. Sci. Sports Exerc. 28:i-vii.
Arieff, A.I. (1986). Hyponatremia, convulsions, respiratory arrest, and permanent brain damage in healthy women. N. Engl. J. Med. 314:1529-1535.
Armstrong, L.E., W.C. Curtis, R.W. Hubbard, R.P. Francesconi, R. Moore, and E.W. Askew (1993). Symptomatic hyponatremia during prolonged exercise in the heat. Med. Sci. Sports Exerc. 25:543-549.
Ayus, J.C., J.M. Wheeler, and A.I. Arieff (1992). Postoperative hyponatremic encephalopathy in menstruant women. Ann. Intern. Med. 117:891-897.
Ayus, J.C., J. Varon, and A.I. Arieff (2000). Hyponatremia, cerebral edema, and noncardiogenic pulmonary edema in marathon runners. Ann. Intern. Med. 132:711-714.
Backer, H.D., E. Shopes, and S.L. Collins (1993). Hyponatremia in recreational hikers in Grand Canyon National Park J. Wilderness Med. 4:391-406.
Craig, S.C. (1999). Hyponatremia associated with heat stress and excessive water consumption: The impact of education and a new Army fluid replacement policy. MSMR 5:1-9.
Davis, D.P., J.S. Videen, A. Marino, G.M. Vike, J.V. Dunford, S.P. Van Camp, and L.G. Maharam (2001). Exercise-associated hyponatremia in marathon runners: a two-year experience. J Emerg. Med. 21:47-57.
Davis, P.B. (2001). Cystic fibrosis. Ped. in Review 22:257-264.
Eichner, E.R. (2002). Exertional hyponatremia: why so many women? Sports Med. Digest 24:54-56.
Freund, B.J., S.J. Montain, A.J. Young, M.N. Sawka, J.P. DeLuca, K.B. Pandolf, and C.R. Valeri (1995). Glycerol hyperhydration: hormonal, renal, and vascular fluid responses. J. Appl. Physiol. 79:2069-2077.
Gardner, J.W. (2002a). Death by water intoxication. Military Med. 5:432-434.
Gardner, J.W. (2002b). Fatal water intoxication of an Army trainee during urine drug testing. Military Med 5:435-437.
Hew, T.D., J.N. Chorley, J.C. Cianca, and J.G. Divine (2003). The incidence, risk factors, and clinical manifestations of hyponatremia in marathon runners. Clin, J. Sports Med. 13:41-47.
Hiller, W.B.D. (1989). Dehydration and hyponatremia during triathlons. Med. Sci. Sports Exerc. 21:5219-5221.
Montain, S.J., M.N. Sawka, and C.B. Wenger (2001). Hyponatremia associated with exercise: risk factors and pathogenesis. Exerc. Sports Sci. Rev. 3:113-117.
Murray, R. (2000). Regulation of fluid balance and temperature during exercise in the heat— scientific and practical considerations. In: H. Nose, C.V. Gisolfi, and K. Imaizumi, (eds.) Exercise, Nutrition, and Environmental Stress. Carmel, IN: Cooper Publishing, pp. 1-20.
National Athletic Training Association (2000). Fluid replacement for athletes. J. Ath. Training 35:212-224.
Noakes, T.D., R.J. Norman, R.H. Buck, J. Godlonton, K. Stevenson, and D. Pittaway (1990). The incidence of hyponatremia during prolonged ultraendurance exercise. Med. Sci. Sports Exerc. 22:165-170.
Noakes, T.D., G. Wilson, D.A. Gray, M.I. Lambert, and S.C. Dennis (2001). Peak rates of diuresis in healthy humans during oral fluid overload. S. African Med. J. 91:852-857.
Noakes, T.D. (2002). Hyponatremia in distance runners: fluid and sodium balance during exercise. Curr. Sports Med. Reports 4:197- 207.
Olsson, K.E. and B. Saltin (1970). Variation in total body water with muscle glycogen changes in man. Acta Physiol. Scand. 80:11- 18, 1970.
O'Toole, M. L., P. S. Douglas, R. H. Laird, and W. D. B. Hiller (1995). Fluid and electrolyte status in athletes receiving medical care at an ultradistance triathlon. Clin. J. Sport Med. 5:116-122.
Pivarnik, J.M., E.M. Leeds, and J.E. Wilkerson (1984). Effects of endurance exercise on metabolic water production and plasma volume. J. Appl. Physiol. 56:613-618.
Quinton, P.M. (1999). Physiological basis of cystic fibrosis: a historical perspective. Physiol. Rev. 79:S3-S22.
Smith, H.R., G.S. Dhatt, W.M. Melia, and J.G. Dickinson (1995). Cystic fibrosis presenting as hyponatraemic heat exhaustion. Br. Med. J. 310:579-580.
Speedy, D.B., T.D. Noakes, T. Boswell, J.M.D. Thompson, N.Rehrer, and D.R. Boswell (2001). Response to a fluid load in athletes with a history of exercise induced hyponatremia. Med. Sci Sports Exerc. 33:1434-1442.
Speedy, D.B., T.D. Noakes, I.R. Rogers, J.M.D. Thompson, R.G.D. Campbell, J.A. Kuttner, D.R. Boswell, S. Wright, and M. Hamlin (1999). Hyponatremia in ultradistance triathletes. Med. Sci. Sports Exerc. 31:809-815.
Zambraski, E.J. (1990). Renal regulation of fluid homeostasis during exercise. In: C.V. Gisolfi and D.R. Lamb (eds.) Perspectives in Exercise Science and Sports Medicine. Vol. 3, Fluid Homeostasis During Exercise. Indianapolis: Benchmark Press, pp. 247-280.
Zehlinger, J., C. Putterman, Y. Ilan, E.J. Dann, F. Zveibel, Y. Shvil, and E. Galun (1996). Case series: hyponatremia associated with moderate exercise. Am. J. Med. Sci. 311:86-91.
VOLUME 16 (2003) NUMBER 1
SUPPLEMENT
Sports Science Exchange 88
DRINKING DO'S AND DON'TS
Dehydration is the most common performance-sapping mistake that athletes make, but it's also the most preventable. Here are some guidelines to help athletes stay well hydrated. Remember that everyone sweats differently and therefore needs to drink a different amount of fluid during exercise.
DO
Start exercise well hydrated
Drink 2-3 cups (475-700 ml) of fluid 2-3 hours before exercise to allow excess fluid to be lost as urine. About one-half hour before exercise, drink 5-10 oz (150-300 ml). There is no benefit to hyperhydration, so don't drink excessively.
Weigh yourself
The best way to determine if you'd had enough to drink during a workout is to check to see how much weight you've lost. Minimal weight loss means that you've done a good job staying hydrated. Remember that weight loss during an exercise session is water loss, not fat loss, and must be replaced.
Drink during exercise
Most athletes find it helpful to drink every 10 to 20 minutes during a workout. Heavy sweaters can benefit from drinking more often (e.g., every 10 minutes) and light sweaters should drink less often (every 20+ minutes).
Ingest sodium during exercise
The best time to begin replacing the sodium lost in sweat is during exercise. That's one reason why a good sports drink is better than plain water.
Follow your own plan
Everybody sweats differently, so everybody needs a drinking plan tailored to his or her individual needs.
Drink plenty during meals
If you weren't able to drink enough during practice to keep from losing weight, be sure to drink enough before the next practice. Mealtime is the best time to do that because of the ease of drinking and the sodium that comes along with food.
DON'T
Don't rely solely on water
Drinking only water keeps you from replacing the electrolytes lost in sweat and from ingesting performance boosting carbohydrates that help you train longer and stronger. Excessive water drinking can lead to dangerous electrolyte disturbances.
Don't overdrink
Water is definitely a good thing, but you can get too much of a good thing. Drinking large amounts of fluid is not only unnecessary, but can be downright dangerous. Bloated stomach, puffy fingers and ankles, a bad headache, and confusion are warning signs of hyponatremia.
Don't gain weight during exercise
A sure sign of drinking too much is weight gain during exercise. If you weigh more after practice than you did before, that means that you drank more than you needed. Be sure to cut back the next time so that you don't gain weight.
Don't restrict salt in your diet
Ample salt (sodium chloride) in the diet is essential to replace the salt lost in sweat. Because athletes sweat a lot, their need for salt is much greater than for non-athletes.
Don't use dehydration to lose weight
Restricting fluid intake during exercise impairs performance and increases the risk of heat-related problems. Dehydration should be kept to a minimum by following a wise fluid-replacement plan.
Don't delay drinking during exercise
Stick to a drinking schedule so that you avoid dehydration early in exercise. Once dehydrated, it's next to impossible to catch up to what your body needs because dehydration actually slows the speed at which fluid exits the stomach.