J. Mark Davis, Ph.D., Adrienne S. Brown, M.A.
CARBOHYDRATES, HORMONES, AND ENDURANCE PERFORMANCE
Sports Science Exchange 80 VOLUME 14 - NUMBER 1
J. Mark Davis, Ph.D. | Adrienne S. Brown, M.A. |
Department of Exercise Science | Department of Exercise Science |
School of Public Health | School of Public Health |
University of South Carolina | University of South Carolina |
Columbia, South Carolina | Columbia, South Carolina |
KEY POINTS
- Prolonged strenuous exercise increases plasma concentrations of the hormones epinephrine, growth hormone, cortisol, and glucagon. Insulin is decreased.
- Ingestion of carbohydrate during prolonged exercise blunts these hormone responses and delays fatigue.
- The blunted hormone response may contribute to a delay in both central (brain) and peripheral (muscle) fatigue by helping to spare liver and muscle glycogen, maintain blood glucose, and reduce blood concentrations of free fatty acids, free tryptophan, and ammonia.
- To prevent a fall in blood glucose concentration and to blunt the hormonal response to exercise, every 15-20 min athletes should drink 8-12 oz (240-350 ml) of a sports drink that contains carbohydrate.
INTRODUCTION
The endocrine (hormonal) system provides for normal bodily functions, including the maintenance of blood glucose levels for optimal health and exercise performance. A decrease in blood glucose during prolonged strenuous exercise can be a major contributor to the onset of fatigue (Davis & Fitts, 1998). The endocrine system attempts to maintain adequate blood glucose levels during exercise by mobilizing other fuels for energy and by stimulating production of glucose from amino acids and other non-carbohydrate sources. Unfortunately, these responses can only delay depletion of the body’s carbohydrate stores, and fatigue can occur in spite of large increases in circulating hormones. In fact, as will be described later, there is some evidence suggesting that the dramatic increase in stress hormones that accompany strenuous exercise may actually hasten fatigue.
Ingesting properly formulated carbohydrate drinks can delay fatigue by keeping blood glucose at high levels (Coggan & Coyle, 1987) and perhaps by sparing muscle glycogen stores (Hargreaves, 2000). Interestingly, the increase in stress-related hormones during exercise is also attenuated when carbohydrate drinks are ingested, but whether this has any bearing on the delay of fatigue is uncertain. Therefore, the purpose of this paper is to briefly review how the glucoregulatory hormones (epinephrine, cortisol, insulin, glucagon, and growth hormone) respond to exercise, how carbohydrate feeding alters these responses, and to judge whether there is an association between altered hormone responses and postponement of fatigue.
RESEARCH REVIEW
Hormonal Response To Prolonged Exercise
At the onset of exercise, nerve impulses from motor centers in the brain (“central command”), along with feedback to the brain’s hypothalamus from sensory nerves originating in muscle, stimulate or inhibit the release of many hormones. Initial rapid changes in hormone secretion occur in anticipation of the need for metabolic and cardiovascular adjustments necessary to support the increased demands imposed by exercise. These hormonal changes become more dramatic as exercise intensity increases and fatigue develops. Hormonal changes may also occur or intensify to support psychological or emotional factors during intense exercise (Galbo, 1992).
One of the most important signals involved in control of the neuroendocrine system is a decrease in blood glucose concentration. This is demonstrated most clearly in exercise studies involving low-carbohydrate diets, fasting, and glucose infusion (Kjaer, 1992; Wasserman & Cherrington, 1996). Strenuous prolonged exercise causes predictable decreases in blood glucose and corresponding increases in concentrations of epinephrine (EPI) (Bailey et al., 1993; Burgess et al., 1991a; Francesconi, 1988; Nieman et al., 1995), cortisol (Burgess et al., 1991a; Nieman et al., 1995; Thuma et al., 1995), glucagon (Galbo, 1992; Mitchell et al., 1990; Wasserman & Cherrington, 1996), and growth hormone (GH) (Murray et al., 1995; Neiman et al., 1998; Utter et al., 1999), along with a decrease in insulin (Burgess et al., 1991a,b; Murray et al., 1991, 1995; Utter et al., 1999; Wasserman & Cherrington, 1996). These hormones have a primary role in maintaining a stable concentration of blood glucose and are often referred to as glucoregulatory hormones (Table 1).
The responses of the glucoregulatory hormones to strenuous prolonged exercise (Figure 1) are more pronounced with increasing exercise duration, i.e., as carbohydrate availability becomes limited and fatigue develops. The rather small changes early in exercise are designed primarily to mobilize extra fuel to meet the increased energy demands of exercise, to shift utilization toward increased fat metabolism, and to maintain blood glucose concentration. The large hormonal changes that occur late in exercise as fatigue develops are caused by the depletion of glycogen in liver and muscle, by the inability to maintain an adequate blood glucose concentration, and by psychological factors related to the increase in effort required to maintain power and to impaired mood state.
TABLE 1. Principal actions of glucoregulatory hormones and some important outcomes of those actions.
Effects Of Carbohydrate Ingestion On Hormonal Responses To Exercise
Carbohydrate ingestion immediately before and/or during endurance exercise produces significant alterations in the gluco regulatory hormones. These responses include a blunting of the typical increases in EPI, cortisol, glucagon, and GH, and a smaller decrease in insulin. Insulin may actually increase with carbohydrate administration during exercise (Figure 2). This response generally supports the premise that maintenance of plasma glucose is a primary role of these hormones during prolonged exercise.
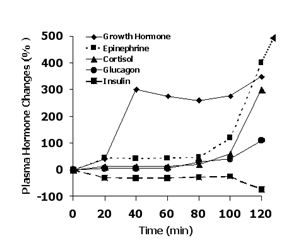
Figure 1
Schematic depiction of changes in plasma glucoregulatory hormone concentrations during 2 h of exercise at 70% VO2max when athletes do not consume carbohydrate during exercise.
Ingestion of 30-60 g of carbohydrate per hour is sufficient to prevent a drop in blood glucose concentration and to delay fatigue during prolonged exercise (Hargreaves, 2000). Hormone studies using similar feeding protocols typically find that the carbohydrate feedings blunt the glucoregulatory hormone response to exercise. In contrast, when subjects are given only 13 g carbohydrate per hour during nearly 3 h of exercise at 70% VO2max, there is no effect on various metabolic variables, ratings of perceived exertion, EPI, cortisol, glucagon, insulin, and time to fatigue (Burgess et al., 1991a).
Insulin. When carbohydrate is ingested during exercise, plasma insulin concentrations are typically maintained at resting levels or in some instances increased (Ahlborg & Felig, 1976; Burgess et al., 1991b; Coyle et al., 1983; Davis et al., 1992; Fritzsche et al., 2000; Murray et al., 1991; Nieman et al., 1998).
Epinephrine (EPI). Carbohydrate ingestion blunts the EPI increase during exercise in most studies (Deuster et al., 1992; Fritzsche et al., 2000; Mitchell et al., 1990; Nieman et al., 1998). In one interesting report, increases in EPI were blunted during 122 min of cycling at 62% VO2max and following 2.5 h of cycling but not running at 75%VO2max (Utter et al. 1999). It is unclear why this exercise mode-specific response occurred.
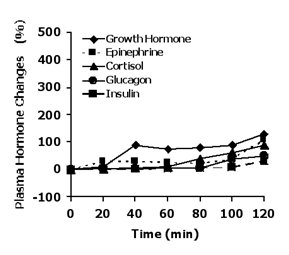
Figure 2
Schematic depiction of changes in plasma glucoregulatory hormone concentrations during 2 h of exercise at 70% VO2max when athletes ingest 30-60 g of carbohydrates during each hour of exercise.
Cortisol. Carbohydrate ingestion during prolonged exercise can also blunt the increase in cortisol concentration during the exercise and for up to several hours thereafter (Nieman et al., 1998; Davis et al., 1989). Utter et al. (1999) showed that cortisol actually decreased following 2.5 h of cycling or running when subjects ingested carbohydrate solutions in comparison to the placebo condition, in which cortisol was maintained at or slightly higher than pre-exercise values. Others have shown similar results during continuous exercise for 2 h (Deuster et al., 1992; Murray et al., 1991, 1995) or following seven 12-min cycling bouts at 70% VO2peak (Mitchell et al., 1990).
Glucagon and Growth Hormone (GH). The increases in blood glucagon and GH during exercise can be attenuated by carbohydrate feedings. Glucose feedings completely blocked the glucagon response to 4 h of cycling at 30% VO2max (Ahlborg & Felig, 1976) but did not affect the glucagon response to intermittent cycling at 70% VO2max (Mitchell et al., 1990). Elevations in plasma GH were blunted following 2.5 h of either cycling or running at 75% VO2max in subjects given carbohydrate drinks versus a water placebo (Nieman et al., 1998; Utter et al., 1999).
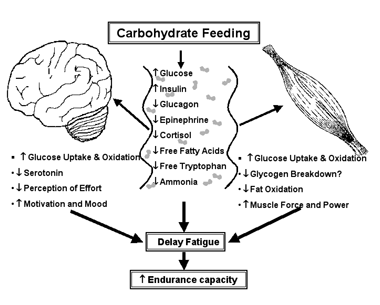
Figure3
Schematic depiction of how consuming carbohydrate-containing sports drinks during prolonged strenuous exercise might delay fatigue by altering fuels (glucose and fatty acids), hormones, and fatigue-related metabolites (free tryptophan and ammonia) in the blood. These changes in the blood can affect the functions of both the brain and the muscles leading to improved athletic performance.
Possible Role Of Glucoregulatory Hormones In The Delay Of Fatigue Associated With Carbohydrate Feedings
The decreased availability of carbohydrate fuel (glycogen and glucose) and the onset of dehydration are the most important limiting factors during endurance exercise, and it is well established that replacing carbohydrate and fluid during exercise by ingesting properly formulated carbohydrate-containing sports drinks will delay fatigue and increase performance. Still, the precise mechanisms responsible for the positive effects of carbohydrate drinks are not fully understood (Davis & Fitts, 1998; Hargreaves, 2000). Coggan and Coyle (1987) suggested that the primary mechanism of delayed fatigue is maintenance of blood glucose and carbohydrate oxidation rates during the latter stages of exercise, at which time muscle glycogen is limited. Carbohydrate ingestion may also spare muscle glycogen in various fiber types during intermittent cycling and running (Hargreaves, 2000). However, it is also possible that the fatigue mechanisms lie within the brain (Davis, 2000; Gandevia, 1999). Carbohydrate feedings can enhance brain function and improve one’s sense of well being during exercise (Davis, 2000), and most people stop exercising or begin to perform poorly because the effort required to keep going is perceived as too great. This large increase in perception of effort during prolonged exercise almost always precedes an inability of the muscle to produce adequate force or power (Gandevia, 1999). Therefore, the benefits of carbohydrate feedings on delaying fatigue may include a reduced sensation of effort, improved motivation, better mood, and reduced inhibition of central motor drive in the uppermost regions of the brain (Davis, 2000; Gandevia, 1999).
We hypothesize that ingesting carbohydrate during exercise helps maintain blood glucose, thereby reducing the blood concentrations of EPI, glucagon, cortisol, and GH, and increasing insulin. These effects of carbohydrate feeding could delay glycogen depletion in muscle and liver, increase glucose uptake and oxidation in muscle and brain, and lower plasma concentrations of free fatty acids (FFA) and ammonia that may contribute to central fatigue. A severe decline in blood glucose (hypoglycemia) at the point of fatigue is a rare occurrence. Thus, glucose availability to the brain is probably not of primary importance in delaying fatigue. However, it is well known that modest decrements in plasma glucose can cause impairments in cognitive function and mood even prior to activation of the glucoregulatory hormone response and before traditional symptoms of hypoglycemia develop (De Feo et al., 1988; Jones et al., 1990; Merbis et al., 1996). The maintenance of blood glucose for the brain may contribute to lower ratings of perceived exertion often observed under these conditions. Utter et al. (1999) recently demonstrated that lower ratings of perceived exertion in subjects consuming carbohydrate drinks were associated with higher carbohydrate oxidation rates, higher blood glucose, higher insulin, and lower cortisol and GH. Lower ratings of perceived exertion have also been observed with glucose infusion during low-intensity exercise (Tabata et al., 1991) and with consumption of carbohydrate drinks during prolonged cycling at 70% VO2max (Burgress et al., 1991b).
The modest decrease in plasma FFA after carbohydrate feedings (resulting from increased insulin and lower EPI, GH, and cortisol) could also help delay central fatigue. How could this work? When the concentration of FFA in blood is reduced, the concentration of free tryptophan also declines. This means that less tryptophan is taken from the blood and converted to serotonin in the brain. Serotonin is thought to promote central fatigue (Davis et al., 1992).
Carbohydrate feedings also lower blood levels of glucagon and cortisol during exercise, and increase insulin. These changes would be expected to decrease ammonia levels in the blood and the brain (Wasserman & Cherrington, 1996); ammonia is toxic to the brain and may also impair muscle metabolism.
SUMMARY
Late stages of prolonged exercise are often associated with large increases in glucoregulatory hormones, which indicate an inability to maintain blood glucose, and these hormonal changes can be an important sign of impending fatigue. The increase in EPI, cortisol, glucagon and GH, along with a reduction in insulin, may contribute to fatigue. Carbohydrate feedings during exercise can blunt these glucoregulatory hormone responses, and, in part, this may be responsible for delayed onset of fatigue. Every 15-20 min during prolonged exercise, athletes should drink 8-12 oz (240-350 ml) of a sports drink containing carbohydrate to replace carbohydrate and fluid. This will prevent a fall in blood glucose and will likely delay fatigue. The delay in fatigue under these circumstances may involve both central and peripheral mechanisms.
REFERENCES
Ahlborg, G., and P. Felig (1976). Influence of glucose ingestion on fuel-hormone response during prolonged exercise. J. Appl. Physiol. 41: 683-688.
Bailey, S.P., J.M. Davis, and E.N. Ahlborn (1993). Neuroendocrine and substrate responses to altered brain 5-HT activity during prolonged exercise to fatigue. J. Appl. Physiol. 74: 3006-3012.
Burgess, W.A., J.M. Davis, W.P. Bartoli, and J.A. Woods (1991a). Failure of low dose carbohydrate feeding to attenuate glucoregulatory hormone responses and improve endurance performance. Int. J. Sport Nutr. 1: 338-352.
Burgess, M.L., R.J. Robertson, J.M. Davis, and J.M. Norris (1991b). RPE, blood glucose, and carbohydrate oxidation during exercise: effects of glucose feedings. Med. Sci. Sports Exerc. 23: 353-359.
Coggan, A.R., and E.F. Coyle (1987). Reversal of fatigue during prolonged exercise by carbohydrate infusion or ingestion. J. Appl. Physiol. 6: 2388-2395.
Coyle, E.F., J.M. Hagberg, B.F. Hurley, W.H. Martin, A.A. Ehsani, and J.O. Holloszy (1983). Carbohydrate feeding during prolonged strenuous exercise can delay fatigue. J. Appl. Physiol. 55: 230-235.
Davis, J.M., V.E. Cokkinides, W.A. Burgess, and W.P. Bartoli (1989). Effects of a carbohydrate-electrolyte drink or water on the stress hormone response to prolonged intense cycling: renin, angiotensin I, aldosterone, ACTH, and cortisol. In: Z. Laron and A. D.Rogal (eds.) Hormones and Sport. New York: Raven Press, pp. 193-204.
Davis, J.M., S.P. Bailey, J.A. Woods, F.J. Galiano, M. Hamilton, and W.P. Bartoli (1992). Effects of carbohydrate feedings on plasma free-tryptophan and branched-chain amino acids during prolonged cycling. Eur. J. Appl. Physiol. 65: 513-519.
Davis, J.M., and R. Fitts (1998). Mechanisms of muscular fatigue. In: J.L. Roitman (ed.) ACSM’s Resource Manual for Guidelines for Exercise Testing and Prescription. Baltimore: Williams & Wilkins, pp. 182-188.
Davis, J.M. (2000). Nutrition, neurotransmitters and central nervous system fatigue. In: R.J. Maughan (ed.) Nutrition in Sport. Oxford: Blackwell Science Ltd, pp. 171-183.
De Feo, P., V. Gallai, G. Mazzotta, G. Crispino, E. Torlone, G. Perriello, M.M. Ventura, F. Santeusanio, P. Brunetti, and G.B. Bolli (1988). Modest decrements in plasma glucose concentration cause early impairment in cognitive function and later activation of glucose counterregulation in the absence of hypoglycemic symptoms in normal man. J. Clin. Invest. 82: 436-444.
Francesconi, R.P. (1988). Endocrinological responses to exercise in stressful environments. In: K.B. Pandolf (ed.), Exercise and Sport Science Reviews, Vol. 16. New York: MacMillan, pp. 255-284.
Fritzsche, R.G., T.W. Switzer, B.J. Hogkinson, S.H. Lee, J.C. Martin, and E.F. Coyle (2000). Water and carbohydrate ingestion during prolonged exercise increase maximal neuromuscular power. J. Appl. Physiol. 88: 730-737.
Galbo, H. (1992). Endocrine factors in endurance. In: R.J. Shephard and P-O. Åstrand (eds.) Endurance in Sport. Oxford:Blackwell Scientific Publications, pp. 116-126.
Gandevia, S.C. (1999). Mind, muscles and motoneurons. J. Sci. Med. Sport. 2: 167-180.
Hargreaves, M. (2000). Carbohydrate replacement during exercise. In: R.J. Maughan (ed.) Nutrition in Sport. Oxford: Blackwell Science Ltd., pp. 112-118.
Jones, T.W, G. McCarthy, W.V. Tamborlane, S. Caprio, E. Roessler, D. Kraemer, K. Starick-Zych, T. Allison, S.D. Boulware, and R.S. Sherwin (1990). Mild hypoglycemia and impairment of brain stem and cortical evoked potentials in healthy subjects. Diabetes. 39: 1550-1555.
Kjaer, M. (1992). Regulation of hormonal and metabolic responses during exercise in humans. In: J.O. Holloszy (ed.), Exercise and Sport Science Reviews, Vol. 20. New York: Williams & Wilkins, pp.161-184.
Merbis, M.A., F.J. Snoek, K. Kane, and R.J. Heine (1996). Hypoglyceamia induces emotional disruption. Patient Educ. Couns. 29: 117-122.
Mitchell, J.B., D.L. Costill, J.A. Houmard, M.G. Flynn, W.J. Fink, and J.D. Beltz (1990). Influence of carbohydrate ingestion on counterregulatory hormones during prolonged exercise. Int. J. Sports Med. 11: 33-36.
Murray, R., G.L. Paul, J.G. Seifert, and D.E. Eddy (1991). Responses to varying rates of carbohydrate ingestion during exercise. Med. Sci. Sports Exerc. 23: 713-718.
Murray, R., W.P. Bartoli, D.E. Eddy, and M.K. Horn (1995). Physiological and performance responses to nicotinic-acid ingestion during exercise. Med. Sci. Sports Exerc. 27: 1057-1062.
Nieman, D.C., J.C. Ahle, D.A. Henson, B.J. Warren, J. Suttles, J.M. Davis, K.S. Buckley, S. Simandle, D.E. Butterworth, O.R. Fagoaga, and S.L. Nehlsen-Cannarella (1995). Indomethacin does not alter natural killer cell response to 2.5 h of running. J. Appl. Physiol. 79: 748-755. Nieman, D.C., S.L. Nehlsen-Cannarella, O.R. Fagoaga, D.A. Henson, A. Utter, J.M. Davis, F. Williams, and D.E. Butterworth (1998). Influence of mode and carbohydrate on the cytokine response to heavy exertion. Med. Sci. Sports Exerc. 30: 671-678.
Tabata, I., F. Ogita, M. Miyachi, and H. Shibayama (1991). Effect of low blood glucose on plasma CRF, ACTH, and cortisol during prolonged physical exercise. J. Appl. Physiol. 71: 1807-1812.
Thuma, J.R., R. Gilders, M. Verdun, and A.B. Loucks (1995). Circadian rhythm of cortisol confounds cortisol responses to exercise: implications for future research. J. Appl. Physiol. 78: 1657-1664.
Utter, A.C., J. Kang, D.C. Nieman, F. Williams, R.J. Robertson, D.A. Henson, J.M. Davis, and D.E. Butterworth (1999). Effect of carbohydrate ingestion and hormonal responses on ratings of perceived exertion during prolonged cycling and running. Eur. J. Appl. Physiol. 80: 92-99.
Wasserman, D.H., and A.D. Cherrington (1996). Regulation of extramuscular fuel sources during exercise. In: L.B. Rowell and J.T. Shepherd (eds.) Handbook of Physiology. New York: Oxford Press, pp. 1036-1074.
The Gatorade Sports Science Institute® was created to provide current information on developments in exercise science, sports nutrition, and sports medicine and to support the advancement of sports science research. For additional information: In the U.S.A. and Canada: 1-800-616-GSSI (4774) Outside the U.S.A.: 847-967-6092 International Online: http://www.gssiweb.com/ Gatorade Sports Science Institute® Fulfillment Agency P.O. Box 75886, Chicago, IL 60675-5886 U.S.A. |
© 2001 Gatorade Sports Science Institute
This article may be reproduced for non-profit, educational purposes only.